Surplus Carbon Drives Allocation and Plant–Soil Interactions
Trends in ecology & evolution(2020)
摘要
Plant growth is normally constrained by nutrients, water or temperature, not photosynthesis, and plants often have surplus carbohydrates.Secondary metabolites are produced in N-limited plants primarily to dispose of surplus carbon, although they may subsequently help reduce browsing damage.Surplus carbohydrates are translocated from leaves and below ground some are discharged via exudates and mycorrhizal fungi.Root exudates contain more of the elements that plants have in surplus, and less of those in short supply.The abundance and type of mycorrhizal fungi is influenced by the amount and composition of surplus carbon in roots.Surplus carbon provides an alternative lens though which to view interactions between plants and soil organisms. Plant growth is usually constrained by the availability of nutrients, water, or temperature, rather than photosynthetic carbon (C) fixation. Under these conditions leaf growth is curtailed more than C fixation, and the surplus photosynthates are exported from the leaf. In plants limited by nitrogen (N) or phosphorus (P), photosynthates are converted into sugars and secondary metabolites. Some surplus C is translocated to roots and released as root exudates or transferred to root-associated microorganisms. Surplus C is also produced under low moisture availability, low temperature, and high atmospheric CO2 concentrations, with similar below-ground effects. Many interactions among above- and below-ground ecosystem components can be parsimoniously explained by the production, distribution, and release of surplus C under conditions that limit plant growth. Plant growth is usually constrained by the availability of nutrients, water, or temperature, rather than photosynthetic carbon (C) fixation. Under these conditions leaf growth is curtailed more than C fixation, and the surplus photosynthates are exported from the leaf. In plants limited by nitrogen (N) or phosphorus (P), photosynthates are converted into sugars and secondary metabolites. Some surplus C is translocated to roots and released as root exudates or transferred to root-associated microorganisms. Surplus C is also produced under low moisture availability, low temperature, and high atmospheric CO2 concentrations, with similar below-ground effects. Many interactions among above- and below-ground ecosystem components can be parsimoniously explained by the production, distribution, and release of surplus C under conditions that limit plant growth. A pervasive practice in studies of carbon (C) allocation (both distribution of plant biomass and C between above- and below-ground parts and between primary and secondary metabolism) is interpreting the observed patterns in terms of optimisation [1.Franklin O. et al.Modeling carbon allocation in trees: a search for principles.Tree Physiol. 2012; 32: 648-666Crossref PubMed Scopus (163) Google Scholar], economic theory [2.Franklin O. et al.Forests trapped in nitrogen limitation – an ecological market perspective on ectomycorrhizal symbiosis.New Phytol. 2014; 203: 657-666Crossref PubMed Scopus (102) Google Scholar,3.Selosse M.-A. Rousset F. The plant-fungal marketplace.Science. 2011; 333: 828-829Crossref PubMed Scopus (52) Google Scholar], or trade-offs between investments and returns of energy, water, and nutrients [4.Vicca S. et al.Fertile forests produce biomass more efficiently.Ecol. Lett. 2012; 15: 520-526Crossref PubMed Scopus (199) Google Scholar,5.Soudzilovskaia N.A. et al.Global patterns of plant root colonization intensity by mycorrhizal fungi explained by climate and soil chemistry.Glob. Ecol. Biogeogr. 2015; 24: 371-382Crossref Scopus (115) Google Scholar]. Similarly, explanations regarding the considerable quantity and variety of C-rich compounds exuded from plant roots and their manifold effects on soil biota commonly assume that these effects on other organisms are the purpose of the fluxes [6.Canarini A. et al.Root exudation of primary metabolites: mechanisms and their roles in plant responses to environmental stimuli.Front. Plant Sci. 2019; 10: 157Crossref PubMed Scopus (185) Google Scholar,7.Xiong D. et al.The effects of warming and nitrogen addition on fine root exudation rates in a young Chinese-fir stand.For. Ecol. Manag. 2020; 458117793Crossref Scopus (7) Google Scholar]. This implies that plants allocate C specifically to gain other resources (such as nutrients or water) and that such responses were selected for during the course of evolution. In both of these interpretations of the flux of C-containing compounds from leaves to roots and soil, C is used as the ‘currency’, with the implicit assumption that the C fixed in photosynthates (see Glossary) is ‘traded’ for other resources. As the currency for plant growth, the assumption persists that the availability of fixed C limits plant growth, despite abundant evidence to the contrary [8.Körner C. Carbon limitation in trees.J. Ecol. 2003; 91: 4-17Crossref Scopus (702) Google Scholar,9.Millard P. et al.Environmental change and carbon limitation in trees: a biochemical, ecophysiological and ecosystem appraisal.New Phytol. 2007; 175: 11-28Crossref PubMed Scopus (190) Google Scholar]. Plants in natural habitats frequently contain high levels of nonstructural carbohydrates (NSCs) [8.Körner C. Carbon limitation in trees.J. Ecol. 2003; 91: 4-17Crossref Scopus (702) Google Scholar,10.Martínez-Vilalta J. et al.Dynamics of non-structural carbohydrates in terrestrial plants: a global synthesis.Ecol. Monogr. 2016; 86: 495-516Crossref Scopus (238) Google Scholar] and do not exhibit a sustained growth response to elevated CO2, unless replete with nutrients and water [11.Körner C. Paradigm shift in plant growth control.Curr. Opin. Plant Biol. 2015; 25: 107-114Crossref PubMed Scopus (294) Google Scholar,12.Jiang M. et al.The fate of carbon in a mature forest under carbon dioxide enrichment.Nature. 2020; 580: 227-231Crossref PubMed Scopus (69) Google Scholar]. Together, these suggest that under natural conditions, plants often have surplus C, relative to other resources such as nutrients or water. A state of surplus C is also consistent with findings from numerous fertiliser-addition experiments that show that plant biomass production in most terrestrial ecosystems is primarily limited by the availability of nutrients – usually nitrogen (N) and/or phosphorus (P) [13.Augusto L. et al.Soil parent material – a major driver of plant nutrient limitations in terrestrial ecosystems.Glob. Chang. Biol. 2017; 23: 3808-3824Crossref PubMed Scopus (119) Google Scholar,14.Harpole W.S. et al.Nutrient co-limitation of primary producer communities.Ecol. Lett. 2011; 14: 852-862Crossref PubMed Scopus (562) Google Scholar]. Plant responses to N or P limitation may actually be activated by the associated increase in shoot carbohydrate concentrations, given the large similarities in gene expression triggered by N and/or P deficiency and high shoot carbohydrate concentrations [15.Hermans C. et al.How do plants respond to nutrient shortage by biomass allocation?.Trends Plant Sci. 2006; 11: 610-617Abstract Full Text Full Text PDF PubMed Scopus (718) Google Scholar]. Under conditions of inadequate availability of N and/or P [16.Assuero S.G. et al.The decrease in growth of phosphorus-deficient maize leaves is related to a lower cell production.Plant Cell Environ. 2004; 27: 887-895Crossref Scopus (96) Google Scholar,17.Kavanová M. et al.Phosphorus deficiency decreases cell division and elongation in grass leaves.Plant Physiol. 2006; 141: 766-775Crossref PubMed Scopus (93) Google Scholar], or water [18.Muller B. et al.Water deficits uncouple growth from photosynthesis, increase C content, and modify the relationships between C and growth in sink organs.J. Exp. Bot. 2011; 62: 1715-1729Crossref PubMed Scopus (441) Google Scholar], plant growth tends to decrease at an earlier stage of limitation than does photosynthesis. This has profound consequences for both leaf C metabolism (Box 1) and C allocation within the plant. Here, we present an alternative view of C allocation that it is largely driven by disposal of surplus C.Box 1Consequences of Nutrient Limitation on Leaf C MetabolismWhen N or P are in short supply, rates of leaf cell division and elongation decline, while photosynthesis continues [16.Assuero S.G. et al.The decrease in growth of phosphorus-deficient maize leaves is related to a lower cell production.Plant Cell Environ. 2004; 27: 887-895Crossref Scopus (96) Google Scholar,17.Kavanová M. et al.Phosphorus deficiency decreases cell division and elongation in grass leaves.Plant Physiol. 2006; 141: 766-775Crossref PubMed Scopus (93) Google Scholar,63.Shi Q. et al.Phosphorus-fertilisation has differential effects on leaf growth and photosynthetic capacity of Arachis hypogaea L.Plant Soil. 2020; 447: 99-116Crossref Scopus (11) Google Scholar], resulting in leaves having surplus photosynthates. The photosynthetic machinery depends on photosynthate removal from the site of synthesis in order to avoid feedback inhibition [11.Körner C. Paradigm shift in plant growth control.Curr. Opin. Plant Biol. 2015; 25: 107-114Crossref PubMed Scopus (294) Google Scholar,64.Sharkey T.D. et al.Limitation of photosynthesis by carbon metabolism: II. O2-insensitive CO2 uptake results from limitation of triose phosphate utilization.Plant Physiol. 1986; 81: 1123-1129Crossref PubMed Google Scholar] and consequent photodamage. In P-deficient plants, photosynthesis produces triose-P, which is converted into sucrose through a series of reactions that liberate inorganic P, which is retained and reused [65.Tuncel A. Okita T.W. Improving starch yield in cereals by over-expression of ADPglucose pyrophosphorylase: Expectations and unanticipated outcomes.Plant Sci. 2013; 211: 52-60Crossref PubMed Scopus (74) Google Scholar]. In leaves of N-deficient plants, surplus photosynthates are converted into sucrose or starch, or shunted into secondary metabolic pathways through which they are converted into C-based secondary metabolites such as flavonoids, terpenoids, hydrolyzable tannins, and phenylpropanoid derivatives [52.Koricheva J. et al.Regulation of woody plant secondary metabolism by resource availability: hypothesis testing by means of meta-analysis.Oikos. 1998; 83: 212-226Crossref Scopus (431) Google Scholar]. During the synthesis of phenylpropanoids, ammonium is released and reused, while the N-free C skeletons of t-cinnamate are shunted into various phenylpropanoid pathways [66.Kováčik J. et al.Phenylalanine ammonia-lyase activity and phenolic compounds accumulation in nitrogen-deficient Matricaria chamomilla leaf rosettes.Plant Sci. 2007; 172: 393-399Crossref Scopus (119) Google Scholar]. Therefore, N-deficient plants have higher concentrations of phenolic compounds than plants subjected to N deposition [52.Koricheva J. et al.Regulation of woody plant secondary metabolism by resource availability: hypothesis testing by means of meta-analysis.Oikos. 1998; 83: 212-226Crossref Scopus (431) Google Scholar]. Elevated concentrations of phenolic compounds such as flavanols, anthocyanins, and coumarins in plants growing with N limitation [67.Pichersky E. Raguso R.A. Why do plants produce so many terpenoid compounds?.New Phytol. 2018; 220: 692-702Crossref PubMed Scopus (126) Google Scholar] are metabolic consequences of plants eliminating surplus photosynthates, while conserving N. Therefore, the primary function of secondary metabolites in N-deficient plants may be to prevent photodamage by removing surplus photosynthates. Leaf secondary metabolites can make foliage less palatable to herbivores, so their production has often been interpreted as C allocation to defend leaves, thereby protecting the plants ability for photosynthesis [68.Juszczuk I.M. et al.Changes in the concentration of phenolic compounds and exudation induced by phosphate deficiency in bean plants (Phaseolus vulgaris L.).Plant Soil. 2004; 267: 41-49Crossref Scopus (79) Google Scholar]. Instead we suggest secondary metabolites are primarily produced for disposal of surplus C, although they may subsequently reduce the risk of browsing damage to the plant. When N or P are in short supply, rates of leaf cell division and elongation decline, while photosynthesis continues [16.Assuero S.G. et al.The decrease in growth of phosphorus-deficient maize leaves is related to a lower cell production.Plant Cell Environ. 2004; 27: 887-895Crossref Scopus (96) Google Scholar,17.Kavanová M. et al.Phosphorus deficiency decreases cell division and elongation in grass leaves.Plant Physiol. 2006; 141: 766-775Crossref PubMed Scopus (93) Google Scholar,63.Shi Q. et al.Phosphorus-fertilisation has differential effects on leaf growth and photosynthetic capacity of Arachis hypogaea L.Plant Soil. 2020; 447: 99-116Crossref Scopus (11) Google Scholar], resulting in leaves having surplus photosynthates. The photosynthetic machinery depends on photosynthate removal from the site of synthesis in order to avoid feedback inhibition [11.Körner C. Paradigm shift in plant growth control.Curr. Opin. Plant Biol. 2015; 25: 107-114Crossref PubMed Scopus (294) Google Scholar,64.Sharkey T.D. et al.Limitation of photosynthesis by carbon metabolism: II. O2-insensitive CO2 uptake results from limitation of triose phosphate utilization.Plant Physiol. 1986; 81: 1123-1129Crossref PubMed Google Scholar] and consequent photodamage. In P-deficient plants, photosynthesis produces triose-P, which is converted into sucrose through a series of reactions that liberate inorganic P, which is retained and reused [65.Tuncel A. Okita T.W. Improving starch yield in cereals by over-expression of ADPglucose pyrophosphorylase: Expectations and unanticipated outcomes.Plant Sci. 2013; 211: 52-60Crossref PubMed Scopus (74) Google Scholar]. In leaves of N-deficient plants, surplus photosynthates are converted into sucrose or starch, or shunted into secondary metabolic pathways through which they are converted into C-based secondary metabolites such as flavonoids, terpenoids, hydrolyzable tannins, and phenylpropanoid derivatives [52.Koricheva J. et al.Regulation of woody plant secondary metabolism by resource availability: hypothesis testing by means of meta-analysis.Oikos. 1998; 83: 212-226Crossref Scopus (431) Google Scholar]. During the synthesis of phenylpropanoids, ammonium is released and reused, while the N-free C skeletons of t-cinnamate are shunted into various phenylpropanoid pathways [66.Kováčik J. et al.Phenylalanine ammonia-lyase activity and phenolic compounds accumulation in nitrogen-deficient Matricaria chamomilla leaf rosettes.Plant Sci. 2007; 172: 393-399Crossref Scopus (119) Google Scholar]. Therefore, N-deficient plants have higher concentrations of phenolic compounds than plants subjected to N deposition [52.Koricheva J. et al.Regulation of woody plant secondary metabolism by resource availability: hypothesis testing by means of meta-analysis.Oikos. 1998; 83: 212-226Crossref Scopus (431) Google Scholar]. Elevated concentrations of phenolic compounds such as flavanols, anthocyanins, and coumarins in plants growing with N limitation [67.Pichersky E. Raguso R.A. Why do plants produce so many terpenoid compounds?.New Phytol. 2018; 220: 692-702Crossref PubMed Scopus (126) Google Scholar] are metabolic consequences of plants eliminating surplus photosynthates, while conserving N. Therefore, the primary function of secondary metabolites in N-deficient plants may be to prevent photodamage by removing surplus photosynthates. Leaf secondary metabolites can make foliage less palatable to herbivores, so their production has often been interpreted as C allocation to defend leaves, thereby protecting the plants ability for photosynthesis [68.Juszczuk I.M. et al.Changes in the concentration of phenolic compounds and exudation induced by phosphate deficiency in bean plants (Phaseolus vulgaris L.).Plant Soil. 2004; 267: 41-49Crossref Scopus (79) Google Scholar]. Instead we suggest secondary metabolites are primarily produced for disposal of surplus C, although they may subsequently reduce the risk of browsing damage to the plant. Growth limitations by N or P do not interfere with the process of phloem loading, so any surplus photosynthates can be exported out of the source leaf to sink organs such as twigs, stems and roots [19.Hammond J.P. White P.J. Sucrose transport in the phloem: integrating root responses to phosphorus starvation.J. Exp. Bot. 2008; 59: 93-109Crossref PubMed Scopus (297) Google Scholar], thereby alleviating the accumulation of fixed C in the leaf and preventing biochemical end-product inhibition and associated phototoxicity. Many nutrient-limited plants accumulate NSCs in roots [20.Kannenberg S.A. Phillips R.P. Plant responses to stress impacts: the C we do not see.Tree Physiol. 2016; 37: 151-153Crossref Scopus (5) Google Scholar,21.Karst J. et al.Stress differentially causes roots of tree seedlings to exude carbon.Tree Physiol. 2016; 37: 154-164Google Scholar]. Accumulation of NSCs stimulates root growth; phloem transport of sucrose and subsequently increased sucrose concentrations in roots are the primary trigger for changes in root metabolism, growth, and gene expression in P-deficient plants [19.Hammond J.P. White P.J. Sucrose transport in the phloem: integrating root responses to phosphorus starvation.J. Exp. Bot. 2008; 59: 93-109Crossref PubMed Scopus (297) Google Scholar]. Over time, this transport of fixed C to roots leads to greater root:shoot ratios of nutrient-limited plants [15.Hermans C. et al.How do plants respond to nutrient shortage by biomass allocation?.Trends Plant Sci. 2006; 11: 610-617Abstract Full Text Full Text PDF PubMed Scopus (718) Google Scholar,22.Lim H. et al.Inter-annual variability of precipitation constrains the production response of boreal Pinus sylvestris to nitrogen fertilization.For. Ecol. Manag. 2015; 348: 31-45Crossref Scopus (54) Google Scholar] or of trees growing on nutrient-poor sites [23.Litton C.M. et al.Carbon allocation in forest ecosystems.Glob. Chang. Biol. 2007; 13: 2089-2109Crossref Scopus (700) Google Scholar]. These phenomena are commonly interpreted as plants adjusting their root: shoot ratio or investing more photosynthates in roots and root symbionts in order to increase nutrient acquisition [4.Vicca S. et al.Fertile forests produce biomass more efficiently.Ecol. Lett. 2012; 15: 520-526Crossref PubMed Scopus (199) Google Scholar,24.Raich J.W. et al.10.16 - Respiration in terrestrial ecosystems.in: Holland H.D. Turekian K.K. Treatise on Geochemistry. 2nd edn. Elsevier, 2014: 613-649Crossref Scopus (11) Google Scholar]. Alternatively, we argue that these shifts in biomass allocation reflect the plant discharging surplus photosynthates belowground when aboveground growth is curtailed by insufficient N or P. The greater flux of photosynthates to roots when aboveground growth is constrained by N or P enhances root exudation (Box 2) and the abundance and growth of root-associated organisms such as ectomycorrhizal (ECM) and arbuscular mycorrhizal fungi [25.Meier I.C. et al.Root exudation of mature beech forests across a nutrient availability gradient: the role of root morphology and fungal activity.New Phytol. 2020; 226: 583-594Crossref PubMed Scopus (14) Google Scholar,26.Ostonen I. et al.Adaptive root foraging strategies along a boreal–temperate forest gradient.New Phytol. 2017; 215: 977-991Crossref PubMed Scopus (57) Google Scholar]. Consistent declines in the abundance of mycorrhizal fungi following additions of N or P [27.Treseder K.K. A meta-analysis of mycorrhizal responses to nitrogen, phosphorus, and atmospheric CO2 in field studies.New Phytol. 2004; 164: 347-355Crossref Scopus (778) Google Scholar] can be attributed to reduced below-ground fluxes of C when nutrient limitation is alleviated. For example, addition of N to a N-limited boreal pine forest resulted in a 60% reduction in below-ground flux of recent photosynthate, and a concomitant reduction in biomass of ECM fungi [28.Högberg M.N. et al.Quantification of effects of season and nitrogen supply on tree below-ground carbon transfer to ectomycorrhizal fungi and other soil organisms in a boreal pine forest.New Phytol. 2010; 187: 485-493Crossref PubMed Scopus (261) Google Scholar]. N addition also causes shifts in relative abundance of fungal taxa. In severely N-limited temperate and boreal forests, ECM fungi dominate, particularly taxa with extensive extramatrical mycelia that form hydrophobic rhizomorphs, and possess proteolytic capacity and potential for organic matter degradation by enzymatic and oxidative activities [29.Lilleskov E.A. et al.Atmospheric nitrogen deposition impacts on the structure and function of forest mycorrhizal communities: a review.Environ. Poll. 2019; 246: 148-162Crossref PubMed Scopus (50) Google Scholar]. N input reduces the abundance of ECM fungi and shifts the community towards short-distance and contact exploration types with much smaller biomass, often with no proteinase activities [29.Lilleskov E.A. et al.Atmospheric nitrogen deposition impacts on the structure and function of forest mycorrhizal communities: a review.Environ. Poll. 2019; 246: 148-162Crossref PubMed Scopus (50) Google Scholar,30.Högberg P. et al.Tamm Review: on the nature of the nitrogen limitation to plant growth in Fennoscandian boreal forests.For. Ecol. Manag. 2017; 403: 161-185Crossref Scopus (79) Google Scholar]. Shifts in arbuscular mycorrhizal fungal associates of plants also occur in response to N addition: from communities dominated by Gigaspora species, which have extensive extramatrical hyphae and possess enzymes capable of degrading recalcitrant compounds under low-N conditions, to dominance by Glomus species, which have minimal extramatrical hyphae and few recalcitrant compound-degrading enzymes [31.Treseder K.K. et al.Arbuscular mycorrhizal fungi as mediators of ecosystem responses to nitrogen deposition: a trait-based predictive framework.J. Ecol. 2018; 106: 480-489Crossref Scopus (60) Google Scholar].Box 2C Surplus and Root ExudationPlants exude a considerable proportion (20–40%) of their assimilated C from their roots [6.Canarini A. et al.Root exudation of primary metabolites: mechanisms and their roles in plant responses to environmental stimuli.Front. Plant Sci. 2019; 10: 157Crossref PubMed Scopus (185) Google Scholar], these root exudates include a wide range of compounds such as simple sugars, amino and organic acids, and a multitude of secondary compounds. Increased flux of photosynthates to roots in nutrient-limited plants can increase rates of exudation. For example, there is a close relationship between the quantity of root exudates and root import of soluble sugars [68.Juszczuk I.M. et al.Changes in the concentration of phenolic compounds and exudation induced by phosphate deficiency in bean plants (Phaseolus vulgaris L.).Plant Soil. 2004; 267: 41-49Crossref Scopus (79) Google Scholar] and a positive linear relationship between sugar concentrations in fine roots and exudation rates of organic C [15.Hermans C. et al.How do plants respond to nutrient shortage by biomass allocation?.Trends Plant Sci. 2006; 11: 610-617Abstract Full Text Full Text PDF PubMed Scopus (718) Google Scholar]. Exudate composition also varies with plant nutritional status; for example, slower release of amino acids from N-depleted plants has been reported for Zea mays (maize) [69.Carvalhais L.C. et al.Root exudation of sugars, amino acids, and organic acids by maize as affected by nitrogen, phosphorus, potassium, and iron deficiency.J. Plant Nutr. Soil Sci. 2011; 174: 3-11Crossref Scopus (288) Google Scholar], Pinus radiata (pine) [70.Bowen G.D. Nutrient status effects on loss of amides and amino acids from pine roots.Plant Soil. 1969; 30: 139-142Crossref Scopus (75) Google Scholar], and Phaseolus vulgaris (bean) [71.Haase S. et al.Elevation of atmospheric CO2 and N-nutritional status modify nodulation, nodule-carbon supply, and root exudation of Phaseolus vulgaris L.Soil Biol. Biochem. 2007; 39: 2208-2221Crossref Scopus (98) Google Scholar]. P limitation increases the release of carbohydrates in Z. mays [69.Carvalhais L.C. et al.Root exudation of sugars, amino acids, and organic acids by maize as affected by nitrogen, phosphorus, potassium, and iron deficiency.J. Plant Nutr. Soil Sci. 2011; 174: 3-11Crossref Scopus (288) Google Scholar] and Gossypium hirsutum (cotton) [72.Yan W.-D. et al.Overexpression of a foreign Bt gene in cotton affects the low-molecular-weight components in root exudates.Pedosphere. 2007; 17: 324-330Crossref Scopus (29) Google Scholar]. The exudation of amino acids by cotton roots also increases under P deficiency [72.Yan W.-D. et al.Overexpression of a foreign Bt gene in cotton affects the low-molecular-weight components in root exudates.Pedosphere. 2007; 17: 324-330Crossref Scopus (29) Google Scholar], consistent with both N and C being available in surplus when P is limiting. Likewise, Glycine max (soybean) roots release more metabolites including amino acids and organic acids when grown with limited P [73.Tawaraya K. et al.Metabolite profiling of soybean root exudates under phosphorus deficiency.Soil Sci. Plant Nutr. 2014; 60: 679-694Crossref Scopus (32) Google Scholar]. Roots may also release a large spectrum of plant secondary metabolites [74.van Dam N.M. Bouwmeester H.J. Metabolomics in the rhizosphere: tapping into belowground chemical communication.Trends Plant Sci. 2016; 21: 256-265Abstract Full Text Full Text PDF PubMed Scopus (246) Google Scholar,75.Strehmel N. et al.Profiling of secondary metabolites in root exudates of Arabidopsis thaliana.Phytochemistry. 2014; 108: 35-46Crossref PubMed Scopus (103) Google Scholar], particularly when plants are nutrient limited [76.Wang Y. et al.Environmental behaviors of phenolic acids dominated their rhizodeposition in boreal poplar plantation forest soils.J. Soils Sedim. 2016; 16: 1858-1870Crossref Scopus (18) Google Scholar,77.Adamczyk B. et al.Plant secondary metabolites – missing pieces in the soil organic matter puzzle of boreal forests.Soil Syst. 2018; 2: 2Crossref Scopus (13) Google Scholar]. Increased concentrations of phenolic compounds [68.Juszczuk I.M. et al.Changes in the concentration of phenolic compounds and exudation induced by phosphate deficiency in bean plants (Phaseolus vulgaris L.).Plant Soil. 2004; 267: 41-49Crossref Scopus (79) Google Scholar], flavanoids [78.Cesco S. et al.Plant-borne flavonoids released into the rhizosphere: impact on soil bio-activities related to plant nutrition. A review.Biol. Fert. Soils. 2012; 48: 123-149Crossref Scopus (191) Google Scholar], and organic acids [79.Jones D.L. Organic acids in the rhizosphere – a critical review.Plant Soil. 1998; 205: 25-44Crossref Scopus (1790) Google Scholar] have been reported in root exudates from nutrient-deficient plants. These differences in exudate profiles are consistent with plants discharging compounds containing the elements that they have in surplus, while retaining those in short supply. There are situations in which specific compounds are produced and exuded in order to produce a specific effect. For example, cluster or dauciform roots that release carboxylates in an exudative burst when P availability is low [80.Shane M.W. et al.Developmental physiology of cluster-root carboxylate synthesis and exudation in harsh hakea. Expression of phosphoenolpyruvate carboxylase and the alternative oxidase.Plant Physiol. 2004; 135: 549-560Crossref PubMed Scopus (104) Google Scholar]. Here, carboxylate exudation is probably a way to acquire P, rather than a way to dispose of surplus C. However, roots may also release carboxylates with a high availability of P [81.Wen Z. et al.Contrastingly patterns in biomass allocation, root morphology and mycorrhizal symbiosis for phosphorus acquisition among 20 chickpea genotypes differing in amount of rhizosheath carboxylates.Funct. Ecol. 2020; 34: 1311-1324Crossref Scopus (9) Google Scholar,82.Huang G. et al.Peppermint trees shift their phosphorus-acquisition strategy along a strong gradient of plant-available phosphorus by increasing their transpiration.Oecologia. 2017; 185 (487-400)Crossref PubMed Scopus (20) Google Scholar]; in such cases, carboxylate exudation may be a way to remove surplus C. Plants exude a considerable proportion (20–40%) of their assimilated C from their roots [6.Canarini A. et al.Root exudation of primary metabolites: mechanisms and their roles in plant responses to environmental stimuli.Front. Plant Sci. 2019; 10: 157Crossref PubMed Scopus (185) Google Scholar], these root exudates include a wide range of compounds such as simple sugars, amino and organic acids, and a multitude of secondary compounds. Increased flux of photosynthates to roots in nutrient-limited plants can increase rates of exudation. For example, there is a close relationship between the quantity of root exudates and root import of soluble sugars [68.Juszczuk I.M. et al.Changes in the concentration of phenolic compounds and exudation induced by phosphate deficiency in bean plants (Phaseolus vulgaris L.).Plant Soil. 2004; 267: 41-49Crossref Scopus (79) Google Scholar] and a positive linear relationship between sugar concentrations in fine roots and exudation rates of organic C [15.Hermans C. et al.How do plants respond to nutrient shortage by biomass allocation?.Trends Plant Sci. 2006; 11: 610-617Abstract Full Text Full Text PDF PubMed Scopus (718) Google Scholar]. Exudate composition also varies with plant nutritional status; for example, slower release of amino acids from N-depleted plants has been reported for Zea mays (maize) [69.Carvalhais L.C. et al.Root exudation of sugars, amino acids, and organic acids by maize as affected by nitrogen, phosphorus, potassium, and iron deficiency.J. Plant Nutr. Soil Sci. 2011; 174: 3-11Crossref Scopus (288) Google Scholar], Pinus radiata (pin
更多查看译文
AI 理解论文
溯源树
样例
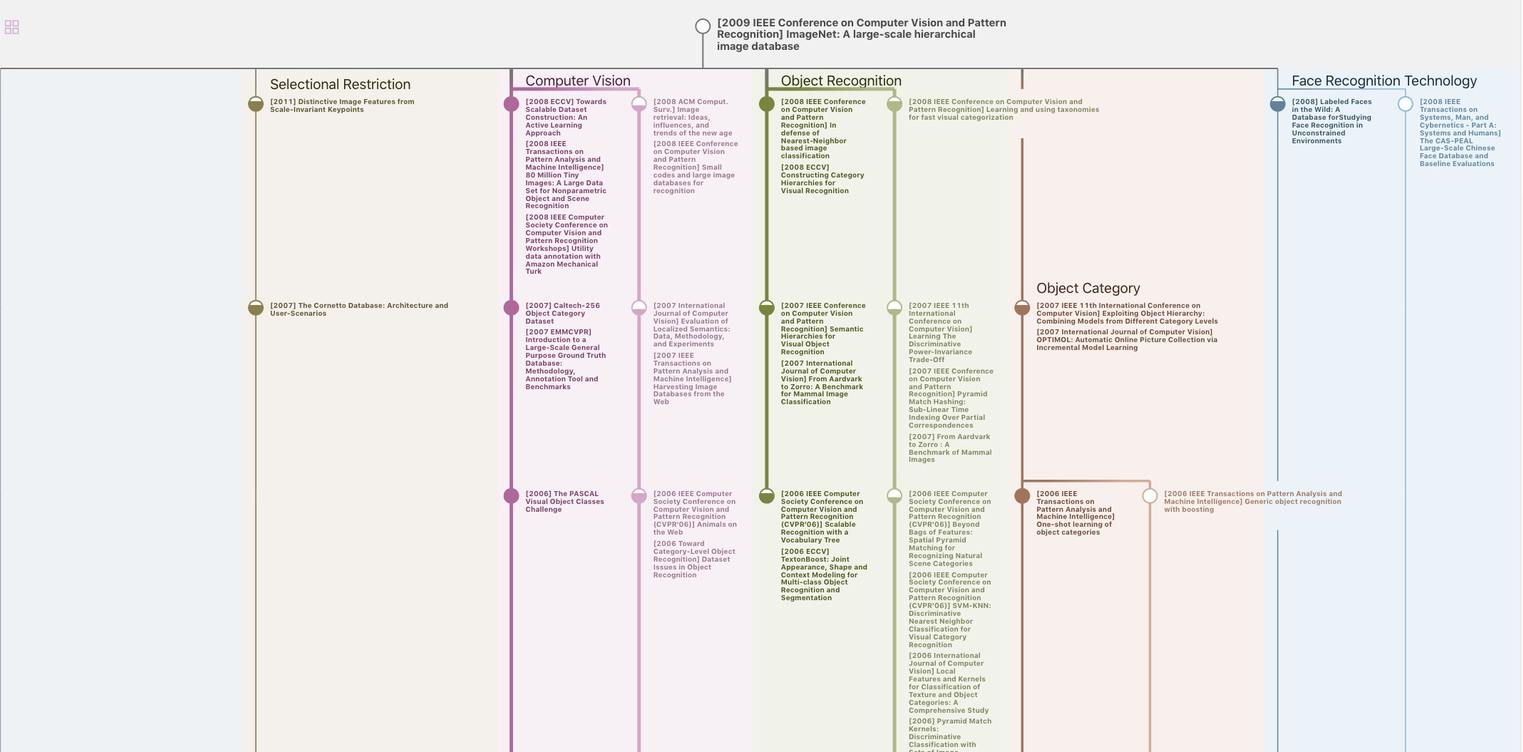
生成溯源树,研究论文发展脉络
Chat Paper
正在生成论文摘要