CHCHD 10 mutations promote loss of mitochondrial cristae junctions with impaired mitochondrial genome maintenance and inhibition of apoptosis
EMBO Molecular Medicine(2015)
摘要
Research Article14 December 2015Open Access Source Data CHCHD10 mutations promote loss of mitochondrial cristae junctions with impaired mitochondrial genome maintenance and inhibition of apoptosis Emmanuelle C Genin Emmanuelle C Genin IRCAN, UMR CNRS 7284/INSERM U1081/UNS, School of Medicine, Nice Sophia-Antipolis University, Nice Cedex 2, France Search for more papers by this author Morgane Plutino Morgane Plutino IRCAN, UMR CNRS 7284/INSERM U1081/UNS, School of Medicine, Nice Sophia-Antipolis University, Nice Cedex 2, France Search for more papers by this author Sylvie Bannwarth Sylvie Bannwarth IRCAN, UMR CNRS 7284/INSERM U1081/UNS, School of Medicine, Nice Sophia-Antipolis University, Nice Cedex 2, France Department of Medical Genetics, National Centre for Mitochondrial Diseases, Nice Teaching Hospital, Nice Cedex 2, France Search for more papers by this author Elodie Villa Elodie Villa INSERM U1065, Centre Méditerranéen de Médecine Moléculaire (C3M), équipe “contrôle métabolique des morts cellulaires”, Nice Sophia-Antipolis University, Nice Cedex 2, France Search for more papers by this author Eugenia Cisneros-Barroso Eugenia Cisneros-Barroso Research Health Institute of Palma (IdISPa), Research Unit, Son Espases University Hospital, Palma de Mallorca, Spain Search for more papers by this author Madhuparna Roy Madhuparna Roy Department of Cell Biology, Johns Hopkins University School of Medicine, Baltimore, MD, USA Search for more papers by this author Bernardo Ortega-Vila Bernardo Ortega-Vila Research Health Institute of Palma (IdISPa), Research Unit, Son Espases University Hospital, Palma de Mallorca, Spain Search for more papers by this author Konstantina Fragaki Konstantina Fragaki IRCAN, UMR CNRS 7284/INSERM U1081/UNS, School of Medicine, Nice Sophia-Antipolis University, Nice Cedex 2, France Department of Medical Genetics, National Centre for Mitochondrial Diseases, Nice Teaching Hospital, Nice Cedex 2, France Search for more papers by this author Françoise Lespinasse Françoise Lespinasse IRCAN, UMR CNRS 7284/INSERM U1081/UNS, School of Medicine, Nice Sophia-Antipolis University, Nice Cedex 2, France Search for more papers by this author Estefania Pinero-Martos Estefania Pinero-Martos Research Health Institute of Palma (IdISPa), Research Unit, Son Espases University Hospital, Palma de Mallorca, Spain Search for more papers by this author Gaëlle Augé Gaëlle Augé IRCAN, UMR CNRS 7284/INSERM U1081/UNS, School of Medicine, Nice Sophia-Antipolis University, Nice Cedex 2, France Department of Medical Genetics, National Centre for Mitochondrial Diseases, Nice Teaching Hospital, Nice Cedex 2, France Search for more papers by this author David Moore David Moore Wellcome Trust Centre for Mitochondrial Research, Institute of Genetic Medicine, International Centre for Life, Newcastle University, Newcastle upon Tyne, UK Newcastle Eye Centre, Royal Victoria Infirmary, Newcastle upon Tyne, UK Search for more papers by this author Florence Burté Florence Burté Wellcome Trust Centre for Mitochondrial Research, Institute of Genetic Medicine, International Centre for Life, Newcastle University, Newcastle upon Tyne, UK Newcastle Eye Centre, Royal Victoria Infirmary, Newcastle upon Tyne, UK Search for more papers by this author Sandra Lacas-Gervais Sandra Lacas-Gervais Joint Center for Applied Electron Microscopy, Nice Sophia-Antipolis University, Nice Cedex 2, France Search for more papers by this author Yusuke Kageyama Yusuke Kageyama Department of Cell Biology, Johns Hopkins University School of Medicine, Baltimore, MD, USA Search for more papers by this author Kie Itoh Kie Itoh Department of Cell Biology, Johns Hopkins University School of Medicine, Baltimore, MD, USA Search for more papers by this author Patrick Yu-Wai-Man Patrick Yu-Wai-Man Wellcome Trust Centre for Mitochondrial Research, Institute of Genetic Medicine, International Centre for Life, Newcastle University, Newcastle upon Tyne, UK Newcastle Eye Centre, Royal Victoria Infirmary, Newcastle upon Tyne, UK Search for more papers by this author Hiromi Sesaki Hiromi Sesaki Department of Cell Biology, Johns Hopkins University School of Medicine, Baltimore, MD, USA Search for more papers by this author Jean-Ehrland Ricci Jean-Ehrland Ricci INSERM U1065, Centre Méditerranéen de Médecine Moléculaire (C3M), équipe “contrôle métabolique des morts cellulaires”, Nice Sophia-Antipolis University, Nice Cedex 2, France Search for more papers by this author Cristofol Vives-Bauza Cristofol Vives-Bauza Research Health Institute of Palma (IdISPa), Research Unit, Son Espases University Hospital, Palma de Mallorca, Spain Search for more papers by this author Véronique Paquis-Flucklinger Corresponding Author Véronique Paquis-Flucklinger IRCAN, UMR CNRS 7284/INSERM U1081/UNS, School of Medicine, Nice Sophia-Antipolis University, Nice Cedex 2, France Department of Medical Genetics, National Centre for Mitochondrial Diseases, Nice Teaching Hospital, Nice Cedex 2, France Search for more papers by this author Emmanuelle C Genin Emmanuelle C Genin IRCAN, UMR CNRS 7284/INSERM U1081/UNS, School of Medicine, Nice Sophia-Antipolis University, Nice Cedex 2, France Search for more papers by this author Morgane Plutino Morgane Plutino IRCAN, UMR CNRS 7284/INSERM U1081/UNS, School of Medicine, Nice Sophia-Antipolis University, Nice Cedex 2, France Search for more papers by this author Sylvie Bannwarth Sylvie Bannwarth IRCAN, UMR CNRS 7284/INSERM U1081/UNS, School of Medicine, Nice Sophia-Antipolis University, Nice Cedex 2, France Department of Medical Genetics, National Centre for Mitochondrial Diseases, Nice Teaching Hospital, Nice Cedex 2, France Search for more papers by this author Elodie Villa Elodie Villa INSERM U1065, Centre Méditerranéen de Médecine Moléculaire (C3M), équipe “contrôle métabolique des morts cellulaires”, Nice Sophia-Antipolis University, Nice Cedex 2, France Search for more papers by this author Eugenia Cisneros-Barroso Eugenia Cisneros-Barroso Research Health Institute of Palma (IdISPa), Research Unit, Son Espases University Hospital, Palma de Mallorca, Spain Search for more papers by this author Madhuparna Roy Madhuparna Roy Department of Cell Biology, Johns Hopkins University School of Medicine, Baltimore, MD, USA Search for more papers by this author Bernardo Ortega-Vila Bernardo Ortega-Vila Research Health Institute of Palma (IdISPa), Research Unit, Son Espases University Hospital, Palma de Mallorca, Spain Search for more papers by this author Konstantina Fragaki Konstantina Fragaki IRCAN, UMR CNRS 7284/INSERM U1081/UNS, School of Medicine, Nice Sophia-Antipolis University, Nice Cedex 2, France Department of Medical Genetics, National Centre for Mitochondrial Diseases, Nice Teaching Hospital, Nice Cedex 2, France Search for more papers by this author Françoise Lespinasse Françoise Lespinasse IRCAN, UMR CNRS 7284/INSERM U1081/UNS, School of Medicine, Nice Sophia-Antipolis University, Nice Cedex 2, France Search for more papers by this author Estefania Pinero-Martos Estefania Pinero-Martos Research Health Institute of Palma (IdISPa), Research Unit, Son Espases University Hospital, Palma de Mallorca, Spain Search for more papers by this author Gaëlle Augé Gaëlle Augé IRCAN, UMR CNRS 7284/INSERM U1081/UNS, School of Medicine, Nice Sophia-Antipolis University, Nice Cedex 2, France Department of Medical Genetics, National Centre for Mitochondrial Diseases, Nice Teaching Hospital, Nice Cedex 2, France Search for more papers by this author David Moore David Moore Wellcome Trust Centre for Mitochondrial Research, Institute of Genetic Medicine, International Centre for Life, Newcastle University, Newcastle upon Tyne, UK Newcastle Eye Centre, Royal Victoria Infirmary, Newcastle upon Tyne, UK Search for more papers by this author Florence Burté Florence Burté Wellcome Trust Centre for Mitochondrial Research, Institute of Genetic Medicine, International Centre for Life, Newcastle University, Newcastle upon Tyne, UK Newcastle Eye Centre, Royal Victoria Infirmary, Newcastle upon Tyne, UK Search for more papers by this author Sandra Lacas-Gervais Sandra Lacas-Gervais Joint Center for Applied Electron Microscopy, Nice Sophia-Antipolis University, Nice Cedex 2, France Search for more papers by this author Yusuke Kageyama Yusuke Kageyama Department of Cell Biology, Johns Hopkins University School of Medicine, Baltimore, MD, USA Search for more papers by this author Kie Itoh Kie Itoh Department of Cell Biology, Johns Hopkins University School of Medicine, Baltimore, MD, USA Search for more papers by this author Patrick Yu-Wai-Man Patrick Yu-Wai-Man Wellcome Trust Centre for Mitochondrial Research, Institute of Genetic Medicine, International Centre for Life, Newcastle University, Newcastle upon Tyne, UK Newcastle Eye Centre, Royal Victoria Infirmary, Newcastle upon Tyne, UK Search for more papers by this author Hiromi Sesaki Hiromi Sesaki Department of Cell Biology, Johns Hopkins University School of Medicine, Baltimore, MD, USA Search for more papers by this author Jean-Ehrland Ricci Jean-Ehrland Ricci INSERM U1065, Centre Méditerranéen de Médecine Moléculaire (C3M), équipe “contrôle métabolique des morts cellulaires”, Nice Sophia-Antipolis University, Nice Cedex 2, France Search for more papers by this author Cristofol Vives-Bauza Cristofol Vives-Bauza Research Health Institute of Palma (IdISPa), Research Unit, Son Espases University Hospital, Palma de Mallorca, Spain Search for more papers by this author Véronique Paquis-Flucklinger Corresponding Author Véronique Paquis-Flucklinger IRCAN, UMR CNRS 7284/INSERM U1081/UNS, School of Medicine, Nice Sophia-Antipolis University, Nice Cedex 2, France Department of Medical Genetics, National Centre for Mitochondrial Diseases, Nice Teaching Hospital, Nice Cedex 2, France Search for more papers by this author Author Information Emmanuelle C Genin1, Morgane Plutino1, Sylvie Bannwarth1,2, Elodie Villa3, Eugenia Cisneros-Barroso4, Madhuparna Roy5, Bernardo Ortega-Vila4, Konstantina Fragaki1,2, Françoise Lespinasse1, Estefania Pinero-Martos4, Gaëlle Augé1,2, David Moore6,7, Florence Burté6,7, Sandra Lacas-Gervais8, Yusuke Kageyama5, Kie Itoh5, Patrick Yu-Wai-Man6,7, Hiromi Sesaki5, Jean-Ehrland Ricci3, Cristofol Vives-Bauza4 and Véronique Paquis-Flucklinger 1,2 1IRCAN, UMR CNRS 7284/INSERM U1081/UNS, School of Medicine, Nice Sophia-Antipolis University, Nice Cedex 2, France 2Department of Medical Genetics, National Centre for Mitochondrial Diseases, Nice Teaching Hospital, Nice Cedex 2, France 3INSERM U1065, Centre Méditerranéen de Médecine Moléculaire (C3M), équipe “contrôle métabolique des morts cellulaires”, Nice Sophia-Antipolis University, Nice Cedex 2, France 4Research Health Institute of Palma (IdISPa), Research Unit, Son Espases University Hospital, Palma de Mallorca, Spain 5Department of Cell Biology, Johns Hopkins University School of Medicine, Baltimore, MD, USA 6Wellcome Trust Centre for Mitochondrial Research, Institute of Genetic Medicine, International Centre for Life, Newcastle University, Newcastle upon Tyne, UK 7Newcastle Eye Centre, Royal Victoria Infirmary, Newcastle upon Tyne, UK 8Joint Center for Applied Electron Microscopy, Nice Sophia-Antipolis University, Nice Cedex 2, France *Corresponding author. Tel: +33 4 93 37 77 86; Fax: +33 4 93 37 70 33; E-mail: [email protected] EMBO Mol Med (2016)8:58-72https://doi.org/10.15252/emmm.201505496 PDFDownload PDF of article text and main figures. Peer ReviewDownload a summary of the editorial decision process including editorial decision letters, reviewer comments and author responses to feedback. ToolsAdd to favoritesDownload CitationsTrack CitationsPermissions Figures & Info Abstract CHCHD10-related diseases include mitochondrial DNA instability disorder, frontotemporal dementia-amyotrophic lateral sclerosis (FTD-ALS) clinical spectrum, late-onset spinal motor neuropathy (SMAJ), and Charcot–Marie–Tooth disease type 2 (CMT2). Here, we show that CHCHD10 resides with mitofilin, CHCHD3 and CHCHD6 within the “mitochondrial contact site and cristae organizing system” (MICOS) complex. CHCHD10 mutations lead to MICOS complex disassembly and loss of mitochondrial cristae with a decrease in nucleoid number and nucleoid disorganization. Repair of the mitochondrial genome after oxidative stress is impaired in CHCHD10 mutant fibroblasts and this likely explains the accumulation of deleted mtDNA molecules in patient muscle. CHCHD10 mutant fibroblasts are not defective in the delivery of mitochondria to lysosomes suggesting that impaired mitophagy does not contribute to mtDNA instability. Interestingly, the expression of CHCHD10 mutant alleles inhibits apoptosis by preventing cytochrome c release. Synopsis CHCHD10 is found to be part of the MICOS complex. Mutations in CHCHD10, previously associated with ALS, cause MICOS disassembly, loss of mitochondrial cristae, mitochondrial genome repair impairment after oxidative stress, and inhibition of apoptosis. CHCHD10 is found to be a component of the MICOS complex. CHCHD10 mutations disassemble the MICOS complex leading to loss of mitochondrial cristae junctions. Assembly of OXPHOS complexes is impaired leading to respiratory chain deficiency. Nucleoids are disorganized resulting in mtDNA repair impairment after oxidative stress. CHCHD10 mutations do not affect mitophagy. Expression of CHCHD10 mutant alleles prevents cytochrome c release and thus inhibits apoptosis via the caspase-dependent pathway. Introduction Seminal studies over the past two decades have identified a growing list of nuclear-encoded mitochondrial genes linked to mitochondrial DNA (mtDNA) instability, either due to mtDNA depletion syndrome or disorders characterized by multiple deletions (Copeland & Longley, 2014). It was predictable that nuclear genes involved in mtDNA replication and repair, or the maintenance of the intramitochondrial nucleotide pool would be implicated in human disorders characterized by mtDNA instability. However, the link between disturbed mitochondrial dynamics and human disease only became apparent with the description of the varied neurological manifestations associated with MFN2 and OPA1 mutations (Rouzier et al, 2012; Burté et al, 2015). More recently, we identified CHCHD10 as a new gene responsible for mtDNA instability disorder (Bannwarth et al, 2014). We described a novel heterozygous CHCHD10 mutation (c.176C>T; p.Ser59Leu) in a large French family with a phenotype including cognitive decline resembling frontotemporal dementia (FTD), motor neuron disease (MND), cerebellar ataxia, and mitochondrial myopathy with multiple mtDNA deletions. The association of FTD with MND in this family led us and others to sequence CHCHD10 in cohorts of patients with frontotemporal dementia-amyotrophic lateral sclerosis (FTD-ALS) or with pure familial or sporadic ALS. Fascinatingly, CHCHD10 mutations were identified in these independent cohorts, firmly establishing a pathophysiological link with FTD-ALS clinical spectrum (Chaussenot et al, 2014; Johnson et al, 2014; Müller et al, 2014; Chiò et al, 2015; Kurzwelly et al, 2015; Ronchi et al, 2015; Zhang et al, 2015). Furthermore, Penttilä and colleagues identified a founder mutation in CHCHD10 (c.197G>T; p.Gly66Val) in 17 Finnish families with late-onset spinal motor neuropathy (SMAJ) (Penttilä et al, 2015), and this variant is also responsible for Charcot–Marie–Tooth disease type 2 (CMT2) (Auranen et al, 2015). CHCHD10 encodes a mitochondrial protein located in the intermembrane space and enriched at cristae junctions but the role played by this protein in both normal and disease states has not yet been established (Bannwarth et al, 2014). Here, we show that CHCHD10 is a component of the mitochondrial contact site and cristae organizing system (MICOS) complex (Pfanner et al, 2014). The expression of CHCHD10 mutant alleles leads to MICOS complex disassembly, loss of mitochondrial cristae, and nucleoid disorganization leading to a defect in mtDNA repair after oxidative stress. Interestingly, the expression of CHCHD10 mutant alleles inhibits apoptosis by preventing cytochrome c release. Our findings support previous studies suggesting that, in some ALS models, motor neuron death can occur via caspase-independent apoptotic mechanisms. Results CHCHD10 is a component of the MICOS complex that is destabilized in CHCHD10 mutant fibroblasts We previously found that CHCHD10 was enriched in the vicinity of mitochondrial cristae junctions as reported for mitofilin, a major component of the MICOS complex (Jans et al, 2013). In order to determine whether CHCHD10 is a component of MICOS, we performed blue native PAGE analysis followed by 2D Western blotting. Results in mouse brain demonstrated that CHCHD10 forms part of a multiprotein complex containing the inner membrane mitochondrial proteins mitofilin, CHCHD3, and CHCHD6 (Fig 1A). Figure 1. CHCHD10 resides in the same complex as mitofilin, CHCHD3 and CHCHD6 Second dimension of the blue native (BN)-PAGE showing that CHCHD10 migrates with the MICOS protein subunits mitofilin, CHCHD3 and CHCHD6 in isolated mitochondria from mouse brain. BN-PAGE of the MICOS and OXPHOS complexes in control (C) and patient (P1, P2) fibroblasts. Complexes I to IV of OXPHOS were detected with the 39 kDa subunit antibody (CI), 70 kDa subunit antibody (CII), core I antibody (CIII), and cytochrome c oxidase subunit I antibody (CIV). MICOS complex was detected with an anti-mitofilin antibody. Second dimension of the BN-PAGE showing that the steady-state levels of assembled CHCHD10 in MICOS complex are decreased in patient fibroblasts. The dividing lines correspond to gel sections visible in the raw data file. Analysis of OXPHOS supercomplexes in control and patient fibroblasts. BN-PAGE from isolated mitochondria permeabilized with 6 g/g (w/v) of digitonin immunoblotted on PVDF membrane and incubated with the indicated antibodies. SC, supercomplexes I+III2+IVn. The dividing lines correspond to gels sections visible in the raw data file. Source data are available online for this figure. Source Data for Figure 1 [emmm201505496-sup-0002-SDataFig1.pdf] Download figure Download PowerPoint We analyzed the effects of CHCHD10 mutant allele on MICOS complex in fibroblasts from our original family carrying the p.Ser59Leu CHCHD10 mutation (Bannwarth et al, 2014). In our first study, we analyzed fibroblasts from patient V-10 (P1). Fibroblasts from a second patient (IV-3; P2) have since become available and they showed the same pathological abnormalities, namely a multiple respiratory chain deficiency (Appendix Table S1), mitochondrial ultrastructural alterations, and fragmentation of the mitochondrial network (Appendix Fig S1). The expression of matrix-targeted photoactivatable GFP showed that mitochondrial fusion is not inhibited in fibroblasts of this novel patient (Appendix Fig S2). To confirm the absence of fusion defect in patient fibroblasts, we ectopically expressed a dominant negative mutant of the fission protein Drp1 (Drp1K38A) (Smirnova et al, 1998) and found that the control and patient cells similarly elongate mitochondria (Appendix Fig S3). Blue native PAGE followed by 2D Western blotting confirmed that, in human fibroblasts, CHCHD10 resides with mitofilin, CHCHD3, and CHCHD6 in the MICOS complex. We also observed a partial disassembly of the complex in fibroblasts of both patients compared with control cells (Fig 1B and C). The protein levels of assembled CHCHD10 and CHCHD3 were decreased in patient fibroblasts (Fig 1C). BN-PAGE analysis also revealed an impairment of OXPHOS complex IV (CIV) assembly. Consequently, OXPHOS supercomplex formation was impaired in both patients, as evidenced by resolving the small supercomplex III+IV by lauryl maltoside solubilization (Fig 1B) and the large supercomplexes I+III2+IVn (SC) by digitonin solubilization (Fig 1D). We then evaluated whether CHCHD10 could physically interact with mitofilin. We found that CHCHD10 and CHCHD3 were immunoprecipitated by a rabbit polyclonal anti-mitofilin antibody both in control and patient fibroblasts. In reverse experiment, mitofilin was immunoprecipitated by a rabbit polyclonal anti-CHCHD10 antibody in HeLa cells (Fig 2A). Last, we used a proximity ligation assay (PLA Duolink) to analyze in situ the proximity between CHCHD10 and mitofilin comparing control and patient fibroblasts. A similar experimental protocol was applied to study the proximity between CHCHD10 and CHCHD6. PLA experiments resulted in a positive labeling in control cells, but quantitative analysis revealed a significant decrease of dot number in patient fibroblasts (Fig 2B). Taken together, these results show that CHCHD10 is an important component of MICOS complex and that CHCHD10 mutation leads to MICOS complex disassembly. Figure 2. Interaction of CHCHD10 with components of MICOS complex Co-immunoprecipitation (IP) of endogenous mitofilin, CHCHD10, and CHCHD3 in control and patient fibroblasts (1 mg of total extracts was used for each IP (left lower panel) and 200 μg was used for the input (left upper panel)). The same results were found in P2 fibroblasts (not shown). Reverse co-IP experiment in HeLa cells with an antibody against CHCHD10 (right lower panel). 1 mg of total extracts was used for each IP and 200 μg was used for the input (right upper panel). The dividing lines correspond to gel sections visible in the raw data file. Duolink proximity ligation assay between mitofilin and CHCHD10 (upper panels), and CHCHD10 and CHCHD6 (lower panels) in control and patient fibroblasts observed by confocal microscopy. Mitochondria were stained with MitoTracker. Duolink spots per cell were quantified for 30 randomly selected individual cells per each studied fibroblast cell line from two independent experiments. Differences between the cell lines were analyzed by Student's t-test (two-sided): highly significant (***P = 0.0001). Scale bar = 10 μm. Source data are available online for this figure. Source Data for Figure 2 [emmm201505496-sup-0003-SDataFig2.pdf] Download figure Download PowerPoint Decrease in nucleoid number without mtDNA depletion in CHCHD10 mutant fibroblasts CHCHD10 mutations lead to MICOS complex disassembly and loss of mitochondrial cristae. It has been suggested that there may be a threshold for the density of cristae junctions required for the maintenance of nucleoid distribution (Itoh et al, 2013). Based on these observations, we visualized nucleoids in patient cells carrying the p.Ser59Leu mutation to determine whether nucleoid disorganization could be responsible for the defect in mtDNA maintenance that was observed in patient skeletal muscle biopsies (Bannwarth et al, 2014). Immunostaining of control and patient fibroblasts with an anti-DNA antibody revealed a significant decrease of nucleoid number in patient cells compared with control cells (Fig 3A and B). Image analysis revealed no aggregation of nucleoids in patient fibroblasts despite a slight increase of nucleoid surface in cells from the first patient (Fig 3C). The phenotype was confirmed using an anti-TFAM antibody, a main protein component of the nucleoids (Appendix Fig S4). Importantly, total mtDNA content did not decrease in CHCHD10 mutant fibroblasts (Fig 3D). Thus, the reduction of nucleoid number is not related to a reduction in the amount of mtDNA. Furthermore, it does not lead to a decrease in expression level of proteins encoded by mtDNA in patient fibroblasts (Appendix Fig S5). Figure 3. Decrease of nucleoid number without mtDNA depletion in patient fibroblasts A. In control and patient fibroblasts, nucleoids were labeled with an antibody against DNA and mitochondria were stained with MitoTracker. Image analysis was performed by confocal microscopy. Scale bar: 10 μm. B, C. The average number (B) and size (C) of nucleoids, labeled with antibodies against DNA as shown in (A), was quantified for 35 randomly selected individual cells per each studied fibroblast cell line from two independent experiments. Differences between the cell lines were analyzed by Student's t-test (two-sided): highly significant (***P < 0.001). Nucleoid number (B): patient 1 versus control ***P = 0.0001, patient 2 versus control ***P = 0.001. Nucleoid size (C): patient 1 versus control ***P = 0.0001. D. Mitochondrial DNA content in control and patient fibroblasts was quantified by real-time PCR. Data were expressed as ratio between mtDNA and nuclear DNA concentration. Results represent the mean of relative PCR ± SD of three independent experiments. Statistical analyses were performed by Student's t-test (two-sided). Download figure Download PowerPoint Expression of CHCHD10 mutations in HeLa cells also leads to a decrease in nucleoid number without mtDNA depletion To substantiate our findings, we also analyzed nucleoid characteristics in HeLa cells expressing CHCHD10 mutant alleles. Among 94 FTD-ALS patients, we previously identified two unrelated cases who carried a novel missense mutation (c.100C>T; p.Pro34Ser) (Chaussenot et al, 2014), which was also reported in one Italian patient with sporadic ALS (Ronchi et al, 2015). To confirm the pathogenic role of this mutation, we analyzed, first, the effects of overexpression of the pathogenic allele on the morphology of the mitochondrial network. After transfection with either empty vector or the wild-type allele, MitoTracker staining revealed a filamentous network. Overexpression of mutant CHCHD10P34S altered mitochondrial morphology in transfected cells with a significant fragmentation of the network (Appendix Fig S6). We also looked at the mitochondrial morphology by electron microscopy. Contrary to overexpression of the wild-type allele, the overexpression of the CHCHD10P34S mutant led to a marked defect of the mitochondrial cristae maintenance characterized by loss and disorganization of cristae morphology (Appendix Fig S7). These results are strikingly similar to those observed when we expressed the p.Ser59Leu CHCHD10 mutation (Bannwarth et al, 2014). Zhang and colleagues also identified the p.Pro34Ser variant in one Canadian patient with Parkinson's disease and in two cases of Alzheimer's disease among cohorts of 153 and 141 patients, respectively (Zhang et al, 2015), and they suggested that it may be not pathogenic. Our data therefore confirm conclusively the deleterious effect of the p.Pro34Ser variant. Both p.Ser59Leu and p.Pro34Ser mutations were then expressed in HeLa cells. Immunostaining with anti-DNA or anti-TFAM antibodies showed a similar pattern to that found in patient fibroblasts with a reduction of nucleoid number, but with no aggregation (Fig 4A–C; Appendix Fig S8). Furthermore, there was no reduction of mtDNA content in cells overexpressing mutant alleles compared with cells overexpressing the wild-type CHCHD10 protein (Fig 4D). Figure 4. Decrease of nucleoid number without mtDNA depletion in HeLa cells overexpressing the CHCHD10S59L and CHCHD10P34S alleles A. Transfections were performed with empty vector (EV) or vectors encoding wild-type CHCHD10-FLAG (WT), CHCHD10-FLAG (S59L), or CHCHD10-FLAG (P34S) mutants. Mitochondria were stained with MitoTracker. Cells overexpressing wild-type and mutant CHCHD10 were labeled with FLAG antibodies. Nucleoids were visualized with an antiserum against DNA. Image analysis was performed by confocal microscopy. Scale bar: 10 μm. B, C. The average number (B) and size (C) of nucleoids, labeled with antibodies against DNA as shown in (A), was quantified for 35 randomly selected individual cells per each studied cell line from two independent experiments. Differences between the cell lines were analyzed by Student's t-test (two-sided): very significant (**P = 0.0280) or highly significant (***P = 0.0001). D. Mitochondrial DNA content was quantified by real-time PCR. Data were expressed as ratio between mtDNA and nDNA concentration. Results represent the mean of relative PCR ± SD of three independent experiments. Statistical analyses were performed by Student's t-test (two-sided). Download figure Download PowerPoint Alteration of nucleoid organization in CHCHD10 mutant fibroblasts Mitochondrial DNA molecules are thought to be closely associated with the mitochondrial inner membrane and they are included within the insoluble fraction of mitochondria (Kanki et al, 2004). In order to determine whether CHCHD10 mutations alter the organization of nucleoids, we tested the distribution of mtDNA in Nonidet P-40 (NP-40) extraction obtained from the mitochondrial fraction of both control and patient fibroblasts. Ratio analysis of mtDNA amplified by qPCR from the supernatant and from the mitochondrial pellet showed that mtDNA was recovered from the particulate fraction in control cells, whereas mtDNA was partially released into the soluble fraction in patient cells. These results are consistent with a disturbed organization of nucleoids (Fig 5A). Figure 5. Nucleoid disorganization in patient fibroblasts leading to a defect in mtDNA repair under conditions of oxidative stress Total extracts from control (C) fibroblasts and intact isolated mitochondria from control and patient fibroblasts (P1, P2) were analyzed by immunoblotting using antibodies against PCNA (nuclear protein), GAPDH (cytosolic protein), or SMAC (mitochondrial intermembrane space protein) (upper panel). Mitochondria from patient and control fibroblasts were incubated with NP-40 and separated into pellets (P) and supernatants (S). The fractions of each extraction were subjected to Western blot analysis. VDAC and SMAC were used to identify behaviors of well-defined mitochondrial proteins that are integral membrane and solub
更多查看译文
关键词
mitochondrial cristae junctions,impaired mitochondrial genome maintenance,apoptosis,mutations
AI 理解论文
溯源树
样例
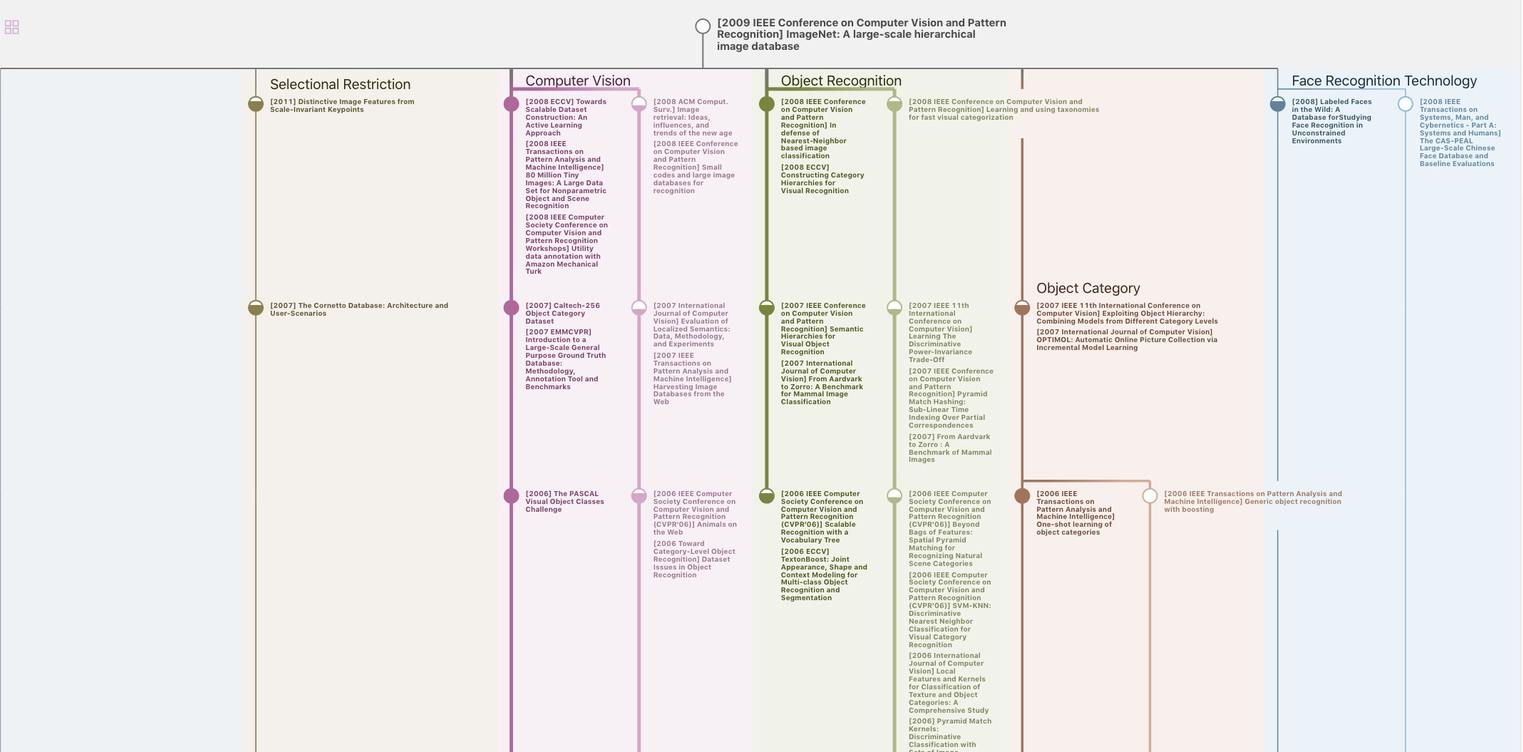
生成溯源树,研究论文发展脉络
Chat Paper
正在生成论文摘要