Scientific practice
semanticscholar(2021)
Abstract
Science is similar to a game, as both involve rules-based participation in search of optimal outcomes. Supported by key texts in history and philosophy of science, the authors propose a game-based model for understanding scientific inquiry and practice, particularly through computational resources. They conclude that this model creates space for more speculative and reflective approaches to scientific practice and can contribute to the design and development of better scientific software, simulation and visualization. In recent decades, the interplay between science and games has intensified. On one hand is an increasing application of video game technology and design into scientific software. Fields such as space exploration, bioinformatics, particle physics, biology and medical imaging take advantage of graphic and interactive capabilities of video game engines, often making use of game design conventions to support data visualization. On the other hand, citizen science games have involved hundreds of thousands of people in scientific inquiry. Gamified citizen science seems to be, so far, the most successful case of Roger Malina’s ideal of “intimate science” [1], connecting the general public to scientific affairs. As a prime example of a citizen science game, Foldit has brought a crowd of users to answer complicated questions on protein folding by engaging them in puzzle-like problem-solving [2]. To a certain extent, both phenomena can be explained by the growth of video games as part of our cultural fabric. Gamification has become a trend in the last decade, having been used by institutions as prestigious as CERN [3] to foster engagement of scientists and enthusiasts in scientific research. Successful interplay between games and science could be explained by their similarities. Games, as described by Marshall McLuhan, were once “live dramatic models of the universe or of the outer cosmic drama” [4], now replaced by scientific models. Moreover, games have been used as a metaphor and analogy for science by scientists and philosophers as diverse as Karl Popper, Thomas Kuhn, Henri Poincaré, Karin Knorr-Cetina and Bruno Latour. That is why we believe that games should be used as a model for understanding, discussing and perfecting certain aspects of scientific practice—particularly when performed through computational resources. Over 30 years ago, Andrew Cunningham used games as a model for science [5]. He was concerned about establishing criteria through which historians of science could confirm whether historical manifestation of scientific practice was, in fact, science (and not, for instance, its spiritual predecessor natural philosophy). Cunningham based his model on a self-elaborated list of typical game features. As we are mainly concerned about scientific software design, we propose a model built upon Jesper Juul’s classic definition of a game: “A game is a rule-based formal system with a variable and quantifiable outcome, where different outcomes are assigned different values, the player exerts effort in order to influence the outcome, the player feels attached to the outcome, and the consequences of the activity are optional and negotiable” [6]. In that case, it is not necessary to specify what kind of game or what kind of science is being discussed, as every science effectively is its own kind of game. To develop such a model, we propose the transposition of each defining trait into scientific terms—a task we carry out through the sections below based on bibliographic investigation on ethnography, philosophy and history of science. Rules and Negotiable Consequences Rules define how a game functions: They are clear, explicit instruction sets about the game’s functioning and players’ actions. Kuhn extensively compared scientific practice to puzzlesolving—two activities with “rules that limit both the nature of acceptable solutions and the steps by which they are to be obtained” [7]. Once transposed to science, the notion of rules could signify at least two different things: methodological rules and scientific laws. Methodological rules include methodologies, methods, stages and processes that must guide scientific research and that support testability, reproducibility and rigor. Methodologies should be guided by logic to ensure science’s integrity. We argue that methodological rules limit and guide the actions of the scientist much as rules of the game guide the player’s actions. Methodological rules are, according to Popper, “the rules of the game of empirical science” [8]. On the other hand, scientific laws are formulated and, eventually, implemented through mathematical models designed to simulate the studied phenomena. Generally speaking, methodological rules guide the actions of scientists, whereas scientific laws govern scientific calculations, models and simulations. Scientific laws can be encoded in a similar way to rules of video games—and not just into software. Gaston Bachelard described scientific instruments as materialized theories in which scientific phenomena take place (rather than in the observable nature) [9]. A similar observation was made by Latour and Steve Woolgar, who emphasized how laboratorial practices are mediated by instruments and equipment that support an “artificial reality” where experiments occur [10]. That takes us to the second defining trait of games: negotiable consequences. Games are usually considered free activities, separate from real life. Johan Huizinga illustrated this idea through the concept of the magic circle, where play takes place isolated and protected from the real world [11]. The laboratory, for authors such as Knorr-Cetina [12] and Mihaly Csikszentmihalyi [13], can be such a place. Additionally, computer simulations—currently the main work environment for many scientists—can make such “artificial reality” feel like a real and productive environment—sometimes dangerously seductive [14]. The separation between science and “reality”—made evident by the way scientific software allows for otherwise impossible experiments—precedes the advent of computational science. Decades before the emergence of computing, Bachelard discussed the mathematization of science, distanced from immediate observation of real-world objects, and moved toward reasoning and ideas. In this case, scientific thought reconstructs reality through “a world created in the image of reason” [15]. This concept—an abstract, rational and fabricated world through which one could understand and reflect on the real world—could easily be used to describe scientific simulation. Variable, Quantifiable and Valued Outcomes Games, Juul argues, should have variable, quantifiable and valued outcomes. Roughly, that means it should be possible for players to achieve different outcomes, measure them and identify those that are more desirable. The goal of science is often identified as a search for truth [16], for the true relationship st a te m en ts 444 LEONARDO, Vol. 54, No. 4, pp. 444–445, 2021 https://doi.org/10.1162/leon_a_02077 ©2021 ISAST Downloaded from http://direct.mit.edu/leon/article-pdf/54/4/444/1959025/leon_a_02077.pdf by guest on 30 August 2021 Statements 445 st a te m en ts between things [17] or for the “ultimate theory” [18]. Scientists’ work can be measured, quantified and valued in at least two ways. First, by comparing experiment results to the expectations set by subjacent theories within their domains. This often requires quantitative data to be gathered, measured and analyzed. Although experiments are usually considered successful when they corroborate subjacent theories or the established standards of their domain, anomalous results could also be valuable, as they could open the way for new scientific paradigms [19]. A second way of measuring outcomes is in the evaluation of scientists’ academic production—theories and experiments—by their peers. For Latour and Woolgar, the production and publication of scientific statements is the main activity taking place at the lab. “The name of the scientific game is to push a statement . . . as far as possible toward fact-like status” [20]. In this case, scientific output can be measured in terms of publications, citations, awards, etc., which, to a certain extent, could determinate one’s sense of recognition (and progression) as a scientist. It seems that in science, as in games, one’s performance and achievements should be measured and evaluated. Effort and Attachment In games, outcomes are usually achieved through players’ efforts. Likewise, scientists operate within the rules of scientific research (often outlined by themselves) to reflect, devise and run experiments—and hope to attain satisfactory results. Such efforts, individual or collective, include the application and combination of intellectual, cognitive and manual/motorsensory skills required for the scientific job involved. In the process, scientists might exercise both logic and intuition [21], convergent and divergent thinking [22], pattern recognition and problem identification and solving skills. Additionally, scientists apply themselves to maintaining a sense of objectivity—often considered the ultimate epistemic virtue [23]—although there is space for more subjective, free and speculative approaches [24]. Moreover, scientists also put effort into constructing scientific statements—usually based on their experiments’ results—that are solid and persuasive enough to be acknowledged by their peers. Indeed, the construction and publication of such statements are of the utmost importance to scientific inquiry [25]. We note the sense of attachment to the outcome. Juul has described it, in players, as a feeling of happiness when winning (or sadness, when losing). In scientific work, achieving successful outcomes might generate a similar sense of satisfaction—either for the intrinsic feeling of “solving a puzzle that no one before has solved” [26] and expanding a field of knowle
MoreTranslated text
AI Read Science
Must-Reading Tree
Example
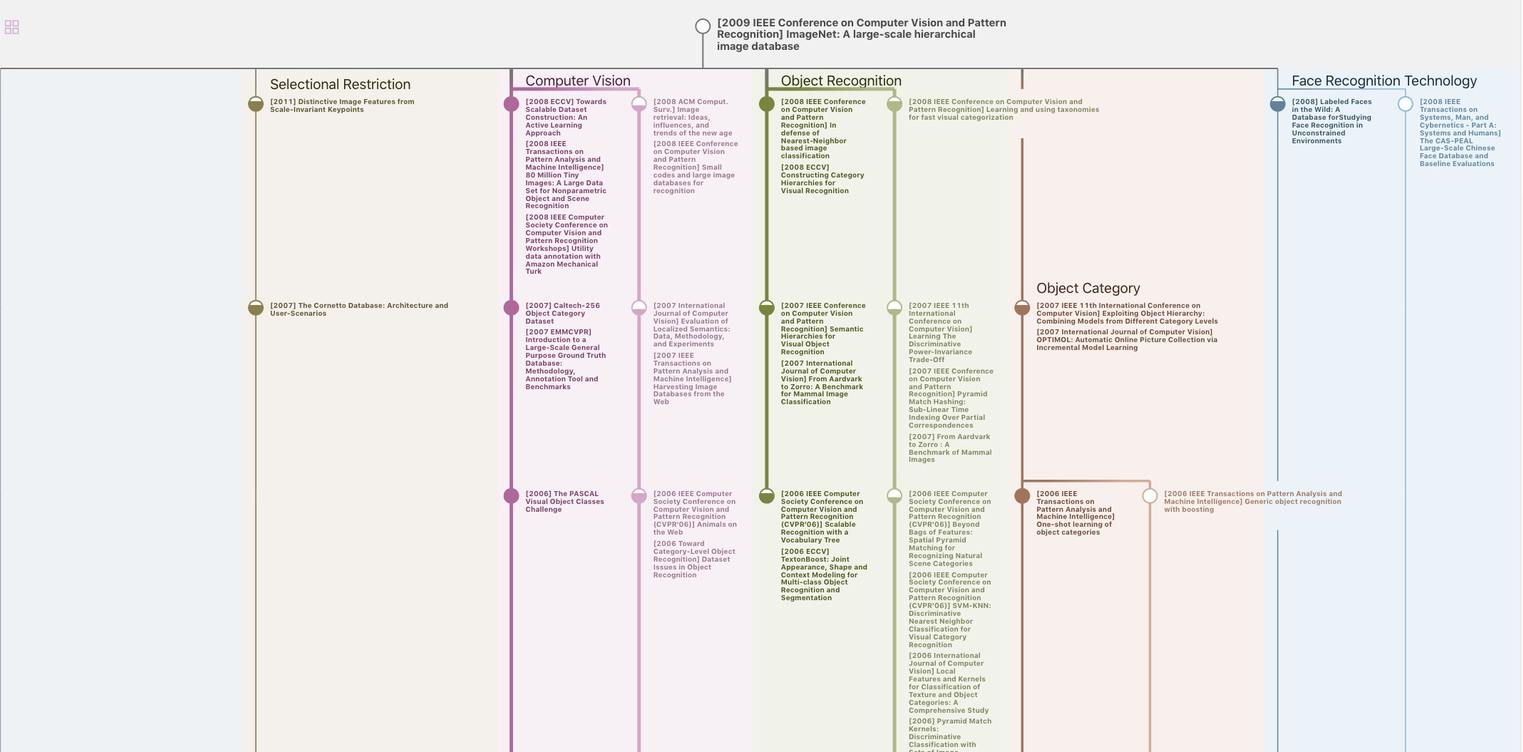
Generate MRT to find the research sequence of this paper
Chat Paper
Summary is being generated by the instructions you defined