The Effect of Space Charge Due to the Auto-Ionization of Neutral, Hydrogenic States in Point-Contact Germanium Detectors at MilliKelvin Temperatures
semanticscholar(2014)
摘要
A class of advanced, semiconducting radiation detectors, when operated at temperatures T~50mK, are able to simultaneously measure both the ionization and phonons created by particle interactions. This has direct application to the search for particle dark matter, as it allows for strong discrimination of the potential signature of dark matter interactions with lattice ions from electromagnetic background. Such detectors currently under research may take the form, for instance, of a P-type Point Contact (PPC) detector. In this work, we explore the effect of space charge accumulation in a germanium PPC detector. This space charge arises due to the autoionization (or field emission) of hydrogenic acceptors subject to high, applied electric fields. At milliKelvin temperatures, the thermionic emission (Fig. 1a) of charge carriers from localized impurities can generally be neglected. Considering relatively large field strengths in this detector (~1000 V/cm), we also neglect the presence of any stable D-/A+ states. We further assume a ptype substrate, dominated by a concentration of acceptors acting as Bohr-like, hydrogenic impurities. In this case, auto-ionization in an external electric field is dominated by quantum mechanical tunneling. Therefore, a bound electron escapes a neutral acceptor from its ground state directly to the continuum (Fig. 1b). Field-dependent ionization rates were calculated by K.M. Sundqvist [2], based on the WKB approximation [3] and implemented using a 3D spatial integral over a neutral acceptor with an assumed isotropic effective mass. We do not model the transport of escaping electrons, but instead use our interpolated ionization rates within COMSOL Multiphysics® software to model a variable density of space charge self-consistently with the electric field. This was performed using the PDE physics interface to model the population of charged acceptors, while the AC/DC Module computed Poisson's equation including space charge (Fig.2). We implemented a logarithmic version of the PDE for the population of charged acceptors, thereby reducing the unphysical affects of large derivatives in our numerical solutions [4]. A 2D axial symmetry was used to model our PPC germanium detector. This included a voltage constant, biased electrode at the point-contact and the entire reverse face held at ground. We find that the space charge created in this detector, even at extremely pure acceptor concentrations of only 5x010 cm−3, still affects the internal electric field (Fig. 3). The effect of bias polarity on the electric field magnitude is also notable (Fig. 4). These results allow us to predict differences in where phonons are produced by drifting carriers, which is important as phonon signals are also measured. These results also lead us to believe that, perhaps with an intentional, regional introduction of the correct impurity type and concentration, we may be able to mitigate unwanted voltage breakdown effects due to high fields near the contact electrodes. Reference [1] N. Mirabolfathi, et al., Neganov–Luke Phonon Amplification in P-type Point Contact Detectors, Journal of Low Temperature Physics, 176, 209, 2014. [2] K.M. Sundqvist, Carrier Transport and Related Effects in Detectors of the Cyrogenic Dark Matter Search, PhD Thesis, University of California, Berkeley, ProQuest/UMI, 2012. [3] D. Bohm, Quantum Theory, Prentice-Hall, Upper Saddle River, NJ, 1951. [4] COMSOL Knowledge Base 952, Avoiding negative concentrations, http://www.comsol.com/ support/knowledgebase/952/. Figures used in the abstract Figure 1: Process a. illustrates classical emission and is exceedingly small in mK operations. Process b. shows the dominant autoionization mechanism in low temperature through quantum mechanical tunneling. Figure 2: AC/DC and PDE coupled physics. NA=Total number of acceptors. Figure 3: Left: Electric field (V/cm) with no space charge, Vb=-400V, Max Efield=36000V/cm; Right: Electric field (V/cm) with space charge, Vb=-400V, NA=5x1010cm−3; Max E-field=26000V/cm. Figure 4: Left: Electric field (V/cm), Vb=+400 V, NA=3x1010cm−3, Max E-field=56000V/cm; Right: Electric field (V/cm), Vb=-400 V, NA=3x1010cm−3, Max E-field=44000V/cm.
更多查看译文
AI 理解论文
溯源树
样例
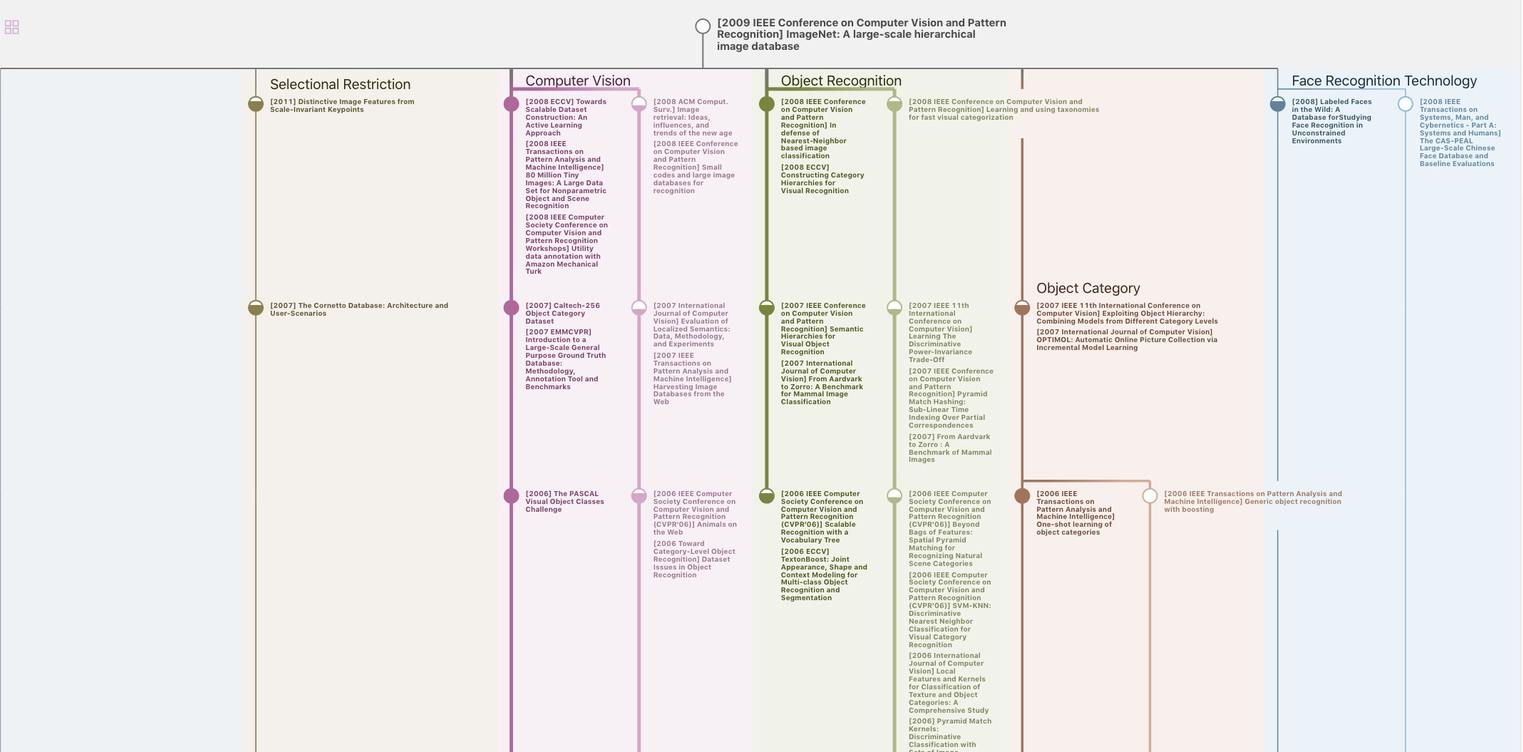
生成溯源树,研究论文发展脉络
Chat Paper
正在生成论文摘要