Analysis of Optical Losses in High-Efficiency CuInS 2-Based Nanocrystal LSCs-Balancing Absorption versus . Scattering
semanticscholar(2018)
摘要
Luminescent solar concentrators (LSCs) use down-converting luminophores embedded in a waveguide to absorb sunlight and deliver high irradiance, narrowband output light for driving photovoltaic and other solar energy conversion devices. Achieving a technologically useful level of optical gain requires bright, broadly absorbing, large-Stokes-shift luminophores incorporated into low-loss waveguides, a combination that has long posed a challenge to the development of practical LSCs. The recent introduction of giant effective Stokes shift semiconductor nanocrystal (NC) phosphors for LSC applications has led to significant performance improvements by increasing solar absorption while reducing escape cone and nonradiative losses compounded by reabsorption, placing increased emphasis on the importance of minimizing parasitic waveguide losses caused by scattering from NC aggregates and optical imperfections. Here, we report a detailed analysis of optical losses in polymer−NC composite waveguide LSCs based on CuInS2/CdS NC phosphors, which have been shown to provide best-in-class performance in largearea, semitransparent concentrators. A comprehensive analytical optical model is introduced enabling quantification of parasitic waveguide, scattering, escape cone, and nonradiative relaxation losses on the basis of distance-dependent edge-emission measurements. By examining the effect of NC loading, we show that NC clustering in polymer composite waveguides leads to light scattering losses that ultimately limit efficiency at large geometric gain. By optimizing NC concentration, optical power efficiencies up to 5.7% under AM1.5 illumination are demonstrated for devices having a geometric gain G = 6.7×, with limiting achievable efficiencies predicted to exceed 10%. ■ INTRODUCTION Solar collectors capable of simultaneous spatial and spectral optical concentration can open new avenues for improving solar conversion efficiency, scalability, and cost by providing high brightness, narrow-bandwidth light optimized for photovoltaic (PV) or photochemical conversion. Luminescent solar concentrators (LSCs), which concentrate light in an inexpensive dielectric waveguide by active frequency shifting, have emerged as one of the most promising approaches for practical solar concentration (Figure 1A). LSCs employ downshifting luminophores to increase the spectral power of photons trapped in a waveguide by total internal reflection, theoretically enabling conversion efficiencies superior to other concentrator designs. PV cells or other converters coupled to the concentrator edges receive intense, bandgap-matched light, while excess energy from above-bandgap light is dissipated over the full area of the LSC. LSCs can concentrate diffuse as well as direct sunlight, are tolerant to partial shading, and can be semitransparent, making them particularly useful for buildingintegrated PV applications such as energy-harvesting window layers. Because some energy is sacrificed in the absorption, downshifting, and transport of sunlight by an LSC, achieving the required high levels of efficiency has long been an obstacle for practical concentrators. The theoretical performance limits of an LSC are established thermodynamically by the optical properties of the luminophores. The upper energy efficiency obeys the single-bandgap Shockley−Queisser limit, and the limiting energy concentration ratio (CRlim) depends on the Stokes shift. For visible to near-IR luminophores, thermodynamically limited theoretical energy concentrations of CRlim > 10 are predicted at room temperature for a Stokes shift of ∼500 meV, corresponding to >10 W m−2 of narrowband output. In practice, however, the performance of LSCs falls far below these thermodynamic limits, mainly because of four Received: December 8, 2016 Revised: January 19, 2017 Published: January 23, 2017 Article
更多查看译文
AI 理解论文
溯源树
样例
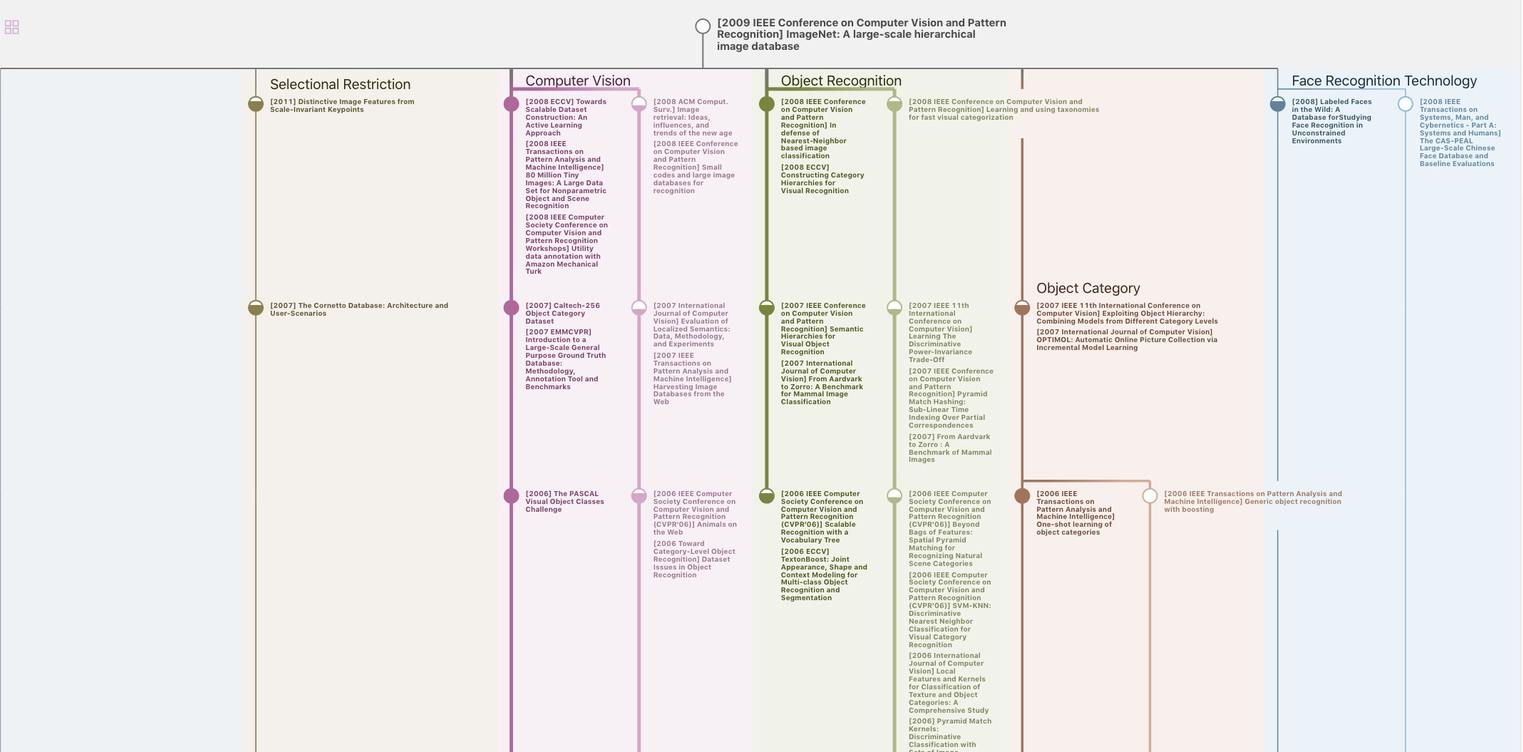
生成溯源树,研究论文发展脉络
Chat Paper
正在生成论文摘要