B 99 , 161113 ( R ) ( 2019 ) Rapid Communications Fragility of Fermi arcs in Dirac semimetals
semanticscholar(2019)
摘要
We use tunable, vacuum ultraviolet laser based angle-resolved photoemission spectroscopy and density functional theory (DFT) calculations to study the electronic properties of Dirac semimetal candidate cubic PtBi 2 . In addition to bulk electronic states we also find surface states in PtBi 2 , which is expected as PtBi 2 was theoretically predicated to be a candidate Dirac semimetal. The surface states are also well reproduced from DFT band calculations. Interestingly, the topological surface states form Fermi contours rather than double Fermi arcs that were observed in Na 3 Bi . The surface bands forming the Fermi contours merge with bulk bands in proximity to the Dirac point projections, as expected. Our data confirm the existence of Dirac states in PtBi 2 and reveal the fragility of the Fermi arcs in Dirac semimetals. Because the Fermi arcs are not topologically protected in general, they can be deformed into Fermi contours, as proposed by M. Kargarian et al. [Proc. Natl. Acad. Sci. USA 113, 8648 (2016)]. Our results demonstrate the validity of this theory in PtBi 2 . Disciplines Condensed Matter Physics Authors Yun Wu, Na Hyun Jo, Lin-Lin Wang, Connor A. Schmidt, Kathryn M. Neilson, Benjamin Schrunk, Przemyslaw Swatek, Andrew Eaton, Sergey L. Bud’ko, Paul C. Canfield, and Adam Kaminski PHYSICAL REVIEW B 99, 161113(R) (2019) Rapid Communications Fragility of Fermi arcs in Dirac semimetals Yun Wu,1,2 Na Hyun Jo,1,2 Lin-Lin Wang,1 Connor A. Schmidt,1,2 Kathryn M. Neilson,1,2 Benjamin Schrunk,1,2 Przemyslaw Swatek,1,2 Andrew Eaton,1,2 S. L. Bud’ko,1,2 P. C. Canfield,1,2,* and Adam Kaminski1,2,† 1Division of Materials Science and Engineering, Ames Laboratory, Ames, Iowa 50011, USA 2Department of Physics and Astronomy, Iowa State University, Ames, Iowa 50011, USA (Received 18 July 2018; published 17 April 2019) We use tunable, vacuum ultraviolet laser based angle-resolved photoemission spectroscopy and density functional theory (DFT) calculations to study the electronic properties of Dirac semimetal candidate cubic PtBi2. In addition to bulk electronic states we also find surface states in PtBi2, which is expected as PtBi2 was theoretically predicated to be a candidate Dirac semimetal. The surface states are also well reproduced from DFT band calculations. Interestingly, the topological surface states form Fermi contours rather than double Fermi arcs that were observed in Na3Bi. The surface bands forming the Fermi contours merge with bulk bands in proximity to the Dirac point projections, as expected. Our data confirm the existence of Dirac states in PtBi2 and reveal the fragility of the Fermi arcs in Dirac semimetals. Because the Fermi arcs are not topologically protected in general, they can be deformed into Fermi contours, as proposed by M. Kargarian et al. [Proc. Natl. Acad. Sci. USA 113, 8648 (2016)]. Our results demonstrate the validity of this theory in PtBi2. DOI: 10.1103/PhysRevB.99.161113 Topological materials hosting Dirac fermions, Weyl fermions, and Majorana fermions are recognized as having the potential to revolutionize the field of high-performance electronics and fault-tolerant quantum computing [1]. The focus on topological materials started with two-dimensional (2D) Dirac states discovered either in 2D materials such as graphene [2] or on the surface of three-dimensional (3D) topological insulators [3–5]. Generalizing the 2D Dirac states to the 3D case leads us to the era of 3D Dirac and Weyl semimetals. Na3Bi and Cd3As2 are the two archetypical 3D Dirac semimetals, where the bulk Dirac points are protected by crystal symmetry [6–8]. Breaking either the time-reversal [9] or inversion symmetry [10] of a 3D Dirac semimetal can result in a Weyl semimetal with pairs of Weyl nodes that have opposite chirality. The Weyl nodes are monopoles in the Berry curvature with nonzero Chern number that naturally lead to exotic surface states such as Fermi arcs. The discovery of Fermi arcs in both type-I [10] and type-II [11–13] Weyl semimetals confirmed the existence of these exotic states. Although Fermi arc surface states have been predicted [14,15] and observed in 3D Dirac semimetals [16,17], the topological nature and stability of these surface states are still under debate [18,19]. Here we demonstrate that the measured surface and bulk band structure of cubic PtBi2 agree well with calculations and confirm that it is a Dirac semimetal. We further demonstrate that the surface state in this system forms closed Fermi contours rather than double Fermi arcs as reported in Na3Bi [16]. Interestingly, the Fermi arcs in Dirac semimetals are not topologically protected; they can be deformed into closed Fermi contours by varying the chemical potential as proposed *canfield@ameslab.gov †kaminski@ameslab.gov by Kargarian et al. [18]. Our data confirm that this indeed occurs in cubic PtBi2 and points to fragility of Fermi arcs in Dirac semimetals due to lack of topological protection (zero chirality of the Dirac nodes). This is contrary to the case in Weyl semimetals, where the open Fermi arc is robust because of the nonzero chirality of the Weyl nodes. PtBi2 crystallizes in at least four phases, one of which is a pyrite type with simple cubic crystal structure (space group 205). The pyrite type PtBi2 was predicted to host 3D Dirac points along the -R line, which are protected by the threefold rotational symmetry [20]. Similarly to some of the topological materials, such as Dirac node arc metal PtSn4 [21,22], Dirac semimetal Cd3As2 [23], and type-II Weyl semimetal WTe2 [24], cubic PtBi2 also exhibits extremely large magnetoresistance up to (11.2×106)% at T = 1.8 K in a magnetic field of 33 T [25]. Interestingly, another phase, hexagonal PtBi2, also exhibits giant magnetoresistance [26]. Topological surface states have been predicted [27] and observed [28,29] in hexagonal PtBi2. However, no evidence of the 3D Dirac semimetallic state has been reported in cubic PtBi2 as of yet. Single crystals of PtBi2 were grown out of Bi-rich binary melts. Elemental Pt and Bi were put into a Canfield crucible set [30] with initial stoichiometry Pt0.05Bi0.95 and sealed into an amorphous silica tube. The ampules were heated up to 430 ◦C within 5 hours, held for 5 hours, cooled to 300 ◦C over 75 hours, and finally decanted using a centrifuge [31]. Single crystals obtained from the growth were ground to obtain a room-temperature powder x-ray diffraction (XRD) pattern with a Rigaku MiniFlex II diffractometer (Cu Kα radiation with monochromator). Band structures with spin-orbit coupling (SOC) in density functional theory (DFT) [32,33] have been calculated using a PBE [34] exchange-correlation functional, a planewave basis set, and projector augmented wave method [35] as implemented in VASP [36,37]. For the bulk band 2469-9950/2019/99(16)/161113(6) 161113-1 ©2019 American Physical Society YUN WU et al. PHYSICAL REVIEW B 99, 161113(R) (2019) FIG. 1. Crystal structure and calculated band structure of cubic PtBi2. (a) Crystal structure of cubic PtBi2 (Pt, white spheres; Bi, red spheres). (b) Brillouin zone of PtBi2. (c) Powder x-ray diffraction (XRD) pattern of PtBi2 (observed pattern, black line; calculated with pyrite structure type [Pa3̄, space group 205], red line; Bi flux peaks, blue stars). (d) Calculated bulk band structure. The red arrow points to the 3D Dirac point along -R line. The green color show the magnitude of the projection on Bi p orbitals. It shows the switching of orbital characters at the bulk Dirac point. (e) Calculated surface Fermi surface at EF with surface Green’s function using a semi-infinite PtBi2 (001) surface with Bi termination. (f) Same as (e) but at EF + 100 meV. The red dots in (e) and (f) mark the projections of the 3D Dirac points in (d). The black arrows point to the surface states (SS). The green arrows mark the spin texture of the surface states. structure of cubic PtBi2, we used the primitive cubic cell of 12 atoms with a Monkhorst-Pack [38] (7×7×7) k-point mesh including the point and a kinetic energy cutoff of 230 eV. The convergence with respect to k-point mesh was carefully checked, with total energy converged below 1 meV/atom. The experimental lattice parameters have been used with atoms fixed in their bulk positions. A tightbinding model based on maximally localized Wannier functions [39–41] was constructed to reproduce closely the bulk band structure including SOC in the range of EF ± 1 eV with Pt sd and Bi p orbitals. Subsequently, the Fermi surface and spectral functions of a semi-infinite PtBi2 (001) surface, with Bi termination, were calculated by using the surface Green’s function methods [42–45] as implemented in WannierTools [46]. Samples used for angle-resolved photoemission spectroscopy (ARPES) measurements were cleaved in situ at 40 K under ultrahigh vacuum. The data were acquired using a tunable vacuum ultraviolet laser ARPES system that consisted of a Scienta R8000 electron analyzer, a picosecond Ti:sapphire oscillator, and a fourth-harmonic generator [47]. Data were collected with a photon energy of 6.7 eV. Momentum and energy resolutions were set at ∼0.005 Å and 2 meV. The size of the photon beam on the sample was ∼30 μm. Figure 1 shows the crystal structure and calculated electronic structure of PtBi2. Figure 1(a) shows the crystal structure of cubic PtBi2, where the red and white spheres correspond to Bi and Pt atoms, respectively. The acquired XRD patterns are well matched with calculated peaks for pyrite structure type of PtBi2 with Pa3̄ (space group 205) as shown in Fig. 1(c). The small-intensity extra peaks marked with blue stars are associated with residual Bi solvent left on the surface of the crystals. Figure 1(d) presents the bulk electronic structure with the red arrow pointing to the 3D Dirac point along the -R line, consistent with the results in Ref. [20]. The coordinates of the bulk Dirac points are ±(0.76, 0.76, 0.76)π/a and at EF − 197 meV [Fig. 1(d)]. The green shad
更多查看译文
AI 理解论文
溯源树
样例
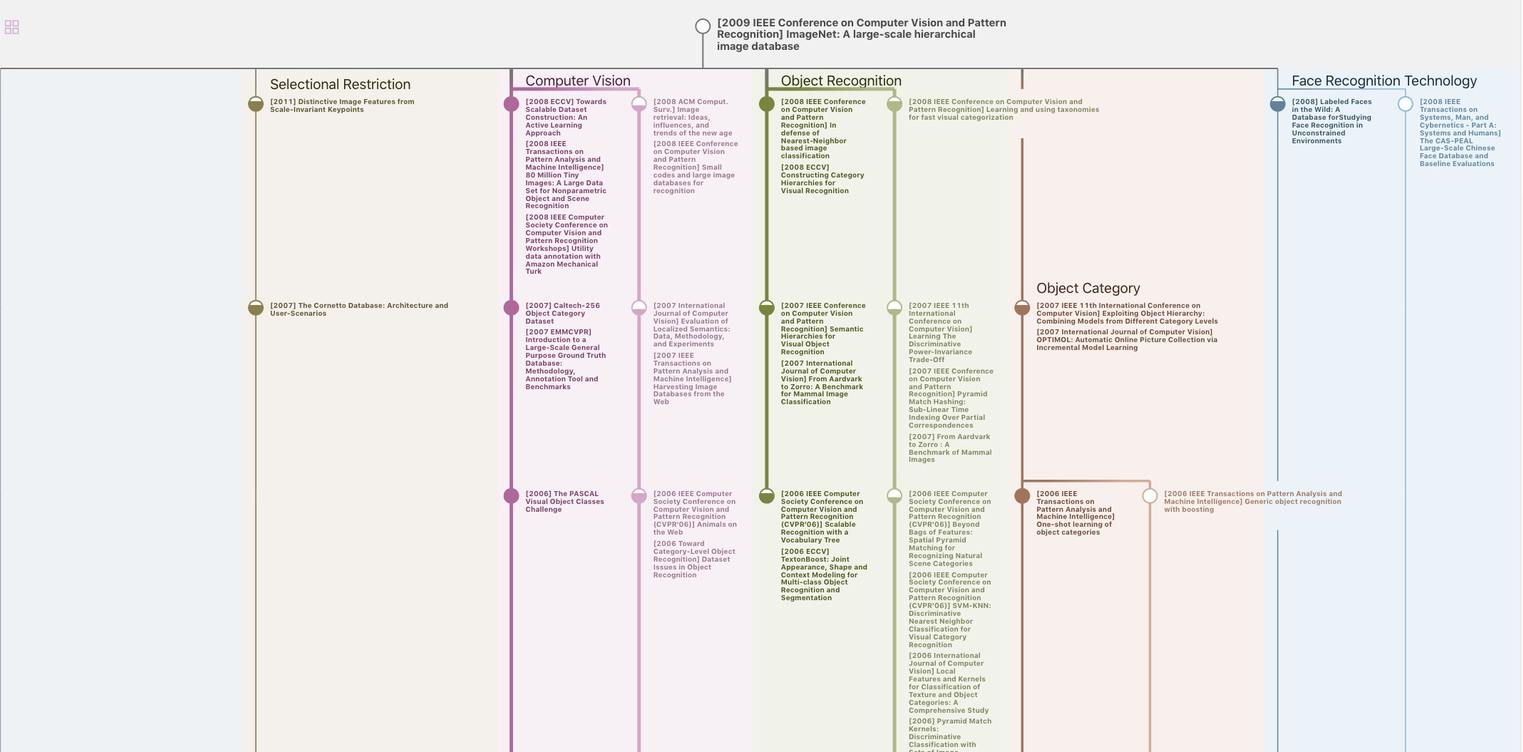
生成溯源树,研究论文发展脉络
Chat Paper
正在生成论文摘要