How Does Zebrafish Support New Strategies for Neuroprotection and Neuroregeneration in Hypoxia-Related Diseases?
Neural regeneration research(2016)
Abstract
Hypoxia is a condition found commonly in several disorders, such as ischemia, asthma, anemia and neonatal hypoxia. Individuals subjected suddenly to high altitude or extreme exercise are also challenged to low oxygen (O2) levels. Since the brain presents elevated basal O2 consumption, this organ is readily affected by hypoxia. For this reason, cerebral hypoxia has been investigated in different species in order to elucidate the involved molecular mechanisms. Based on these models, it is now understood that many of the neural ischemic events occur in cerebral hypoxia, such as excessive release of chelatable Zn from neurons (Udayabanu et al., 2012), glial activation (Li et al., 2010), depletion in mitochondrial activity, increase in reactive oxygen species and cell necrosis (see review in Lukyanova and Kirova, 2015). Moreover, it has been recently reported that chelatable Zn contribute also to the development of the pathophysiology of hypoxia, triggering neuroinflammatory and oxidative stress responses observed in hypoxia (Malairaman et al., 2014). Thus, potential compounds have been tested on hypoxia models to evaluate new neuroregenerative and neuroprotective therapies able to replace the loss of neural cells and decrease the progression of secondary injuries caused by hypoxia, respectively. To advance the research in hypoxia, we have supported the development of a new model in zebrafish. These animals have been used in large scale screening of neuroprotective drugs, because they present features, such as easy maintenance, low operating cost and functional conservation of mammalian brain areas. Moreover, the sharing of many human genes, including those encoding to hypoxia-inducible factors (HIFs), further supports the adoption of zebrafish to evaluate the effects of hypoxia. Zebrafish as model for investigation of mechanisms related to hypoxia resistance: Zebrafish are naturally resistant to hypoxia. Although zebrafish are less tolerant than other fish species, these animals can survive in extremely low levels of O2 compared to normoxic water (~7.5 mg/L O2). The reason for hypoxia tolerance is due to a number of adaptations, such as low critical partial pressure of O2 (PCrit), which maintain aerobic metabolism even under low O2 levels (Barrionuevo and Burggren, 1999). In fact, these features are commonly found in aquatic animals (e.g., turtles and other fish species) or in animals challenged to hypoxia in a stage of life (e.g., insect larvae). In the case of aquatic animals is thought that hypoxia tolerance is an acquired condition due to evolutionary pressures found in aquatic environments, which have a large variation in O2 levels, especially in lakes where the zebrafish naturally survive. Thus, the particular biology of zebrafish has provided the basis for its use in the investigation of mechanisms associated with hypoxia tolerance through open systems (Figure 1), which have supported the evaluation of new therapeutic strategies for treatment of dysfunctions involving low O2 levels. As studies on zebrafish physiology, it is possible that much of its resistance is also associated with a high baseline rate of neurogenesis, an approach which needs to be still investigated in fish subjected to hypoxia.Figure 1: Representation of hypoxia systems used in zebrafish.In the left it is showed a closed system, suitable for the study of new therapeutic strategies for the treatment of cerebral dysfunctions involving low O2 levels. In the right it is showed an open system, used mainly for hypoxia tolerance tests. In this system the O2 from air is available to water.Hypoxia chamber as model to evaluate new therapies in zebrafish: Another application of the model in zebrafish is the screening of drugs with potential action in hypoxia. Indeed, tolerance to hypoxia is an undesirable feature, which could hamper this investigative approach in this species. However, the threshold to hypoxia tolerance may be overcome when they are subjected to hypoxia in closed systems such as a hypoxia chamber (Yu and Li, 2011; Braga et al., 2013a). In this apparatus (Figure 1), adult zebrafish can survive for few minutes (~10 minutes) in water containing less than 2 mg/L O2 (Yu and Li, 2011; Braga et al., 2013). In this condition, known behavioral stages are performed by zebrafish (Braga et al., 2013a), which are associated to brain damages. Considering the characteristics reported of the hypoxia model in closed chamber, it is also noted advantages on other models of diseases involving low levels of O2. Certainly, this model presents unwanted limitations, such as the usual requirement of pooled brains due to small size of samples for investigation of some neurochemical functions. However, in comparison to ischemic models in rodents, hypoxia in fish is obtained through a noninvasive technical procedure, which does yield a low loss of animals during hypoxia trials. Moreover, a wide number of animals may be simultaneously maintained in hypoxia, allowing to evaluate post-hypoxia treatments on fish subjected to same hypoxic assay, which are experimental conditions easily attended only in hypoxia models with invertebrates (e.g., fruit flies). Therefore, hypoxic chamber model in zebrafish consists of quick and practical trials, which are suitable for research on hypoxia mechanisms and on development of new therapies in hypoxia-related diseases. To date, studies from Yu and Li (2011) and from our research group (Braga et al., 2013a) have demonstrated that hypoxia chamber causes impairments on exploratory behavior of adult zebrafish associated with a decrease in brain mitochondrial activity. Additionally, we have reported that abnormal behaviors and mitochondrial function are respectively reverted after 6 hours and 48 hours in normoxic water, indicating the first data about time window for recovering of zebrafish after hypoxia (Braga et al., 2013a). In this same line, we also showed that cerebral region of zebrafish with known high Zn content (Braga et al., 2013b) presented an increase in this metal after 1 hour from hypoxia episode (Braga et al., 2016). We observed still that hypoxia did increase cerebral nitric oxide, superoxide anion and hydroxyl radical, but antioxidant responses (e.g., sulphydryl content, superoxide dismutase activity) remained unaltered after 1 hour from hypoxia trial. Although these first results have demonstrated the sensibility of zebrafish exposed to hypoxia chamber, further studies need to be conducted about the damages developed in short or long term to the hypoxic episode. Diethyldithiocarbamate (DEDTC) has been the first compound used to evaluate a possible protective effect on those neurobehavioral impairments caused by hypoxia in zebrafish. The reasons for research with DEDTC were supported by its Zn chelator action and its ability to cross the blood-brain barrier, though we have observed side effects after per se exposure to high concentrations (in mM range) of DEDTC in zebrafish (Braga et al., 2015). The addition of this compound into water during hypoxic assay increased the survival rate of animals associated with a preventive effect on the decreased mitochondrial activity (Yu and Li, 2013). Also, these authors demonstrated that post-hypoxia exposure of DEDTC prevented the increase of chelatable Zn induced by hypoxia in the brain. By using only post-hypoxia treatment, we observed similar effect of DEDTC in the brain Zn content, but the animals presented impairments on exploratory behaviors and a decline on mitochondrial activity associated with an exacerbate production of radical hydroxyl and induction of antioxidant responses (Braga et al., 2016). The different results between these works remain to be better studied, but different experimental designs related to the treatment period and recovery time may have been responsible for the discrepant outcome of treatment with DEDTC in zebrafish. At the same time, our findings make questionable the role of the chelatable Zn content on the damage caused by hypoxia in the brain. A cerebral eleveation in chelatable Zn level in hypoxia-related models has been reported which is attributed to synaptic Zn release from specific excitatory neurons. Using electronic microscope, we have now located excitatory neurons in the same brain region previously described with high chelatable Zn content in the zebrafish (Figure 2). Thus, future studies may elucidate whether these neurons contain Zn capable of being synaptically released and, if they are responsible in the increase of chelatable Zn content caused by hypoxia in zebrafish.Figure 2: Excitatory neuron located in the optic tectum of zebrafish containing round clear vesicles indicated by arrows.Scale bar: 200 μm. The methodological procedures performed for this sample were based on Hermel et al. (2006).Next steps for research on hypoxia in zebrafish: Because hypoxia chamber model in zebrafish is still a recent model, we have designed our next research by identifying their brain mechanisms concurrently with the screening of new drugs with promising neuroprotective action in this model. To date, we are investigating the effect of an organoselenium compound on hypoxia, which our research group reported recently a modulatory action of this compound on behavior of zebrafish. Moreover, we are evaluating whether hypoxic preconditioning could be beneficial in zebrafish subjected to hypoxia chamber. In fact, the data obtained in these and other studies will may contribute for pre-clinical investigations of these therapies. Since zebrafish has been highlighted in recent years as a model with high neurogenesis, we hope also as soon as possible to investigate the neural regeneration based on generation of new neurons during recovering of hypoxia in the presence of different compounds. We are grateful to the Centro de Microscopia Eletrônica (CME) of the Universidade Federal do Rio Grande do Sul and to Prof. Dr. Maria Cristina Faccioni Heuser for the technical assistance in transmission electronic microscope. This work was supported by Coordenação de Aperfeiçoamento de Pessoal de Nível Superior (CAPES), Conselho Nacional de Desenvolvimento Científico e Tecnológico (CNPq), INCT-Excitoxicidade e Neuroproteção and by FINEP research grant “Rede Instituto Brasileiro de Neurociência (IBN-Net)” #01.06.0842-00.
MoreTranslated text
AI Read Science
Must-Reading Tree
Example
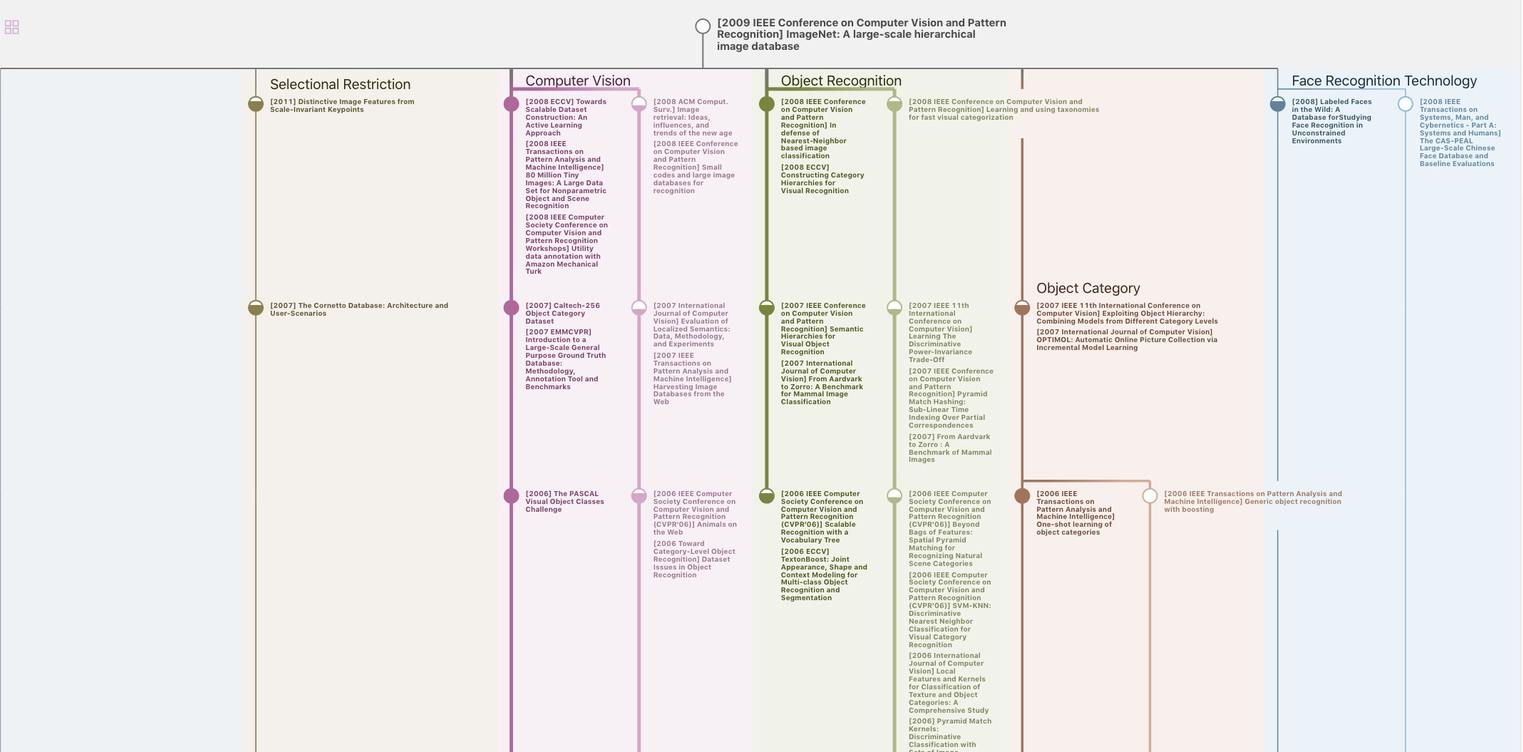
Generate MRT to find the research sequence of this paper
Chat Paper
Summary is being generated by the instructions you defined