Triphasic contrast injection improves evaluation of dual energy lung perfusion in pulmonary CT angiography
European Journal of Radiology(2011)
Abstract
Methods and materials Data of 120 patients who had undergone dual source dual energy CT pulmonary angiography for suspected acute pulmonary embolism were evaluated. Group 1 ( n = 30) was scanned in craniocaudal direction using 64 × 0.6 mm collimation; groups 2–4 ( n = 30 each) were scanned in caudocranial direction using 14 × 1.2 mm collimation. In groups 1–3 biphasic injection protocols with different amounts of CM and NaCl were investigated. In group 4 a split-bolus protocol with an initial CM bolus of 50 ml followed by 30 ml of a 70%:30% NaCl/CM mixture and a 50 ml NaCl chaser bolus was used. CT density values in the subclavian vein (SV), superior vena cava (SVC), pulmonary artery tree (PA), and the descending aorta (DA) were measured. Artifacts arising from the SV and SVC on DE pulmonary iodine distribution map were rated on a scale from 1 to 5 (1 = fully diagnostic; 5 = non-diagnostic) by two blinded readers. Results In protocol 4 mean attenuation in the SV (645 ± 158 HU) and SVC (389 ± 114 HU) were significantly lower compared to groups 1–3 ( p < 0.002). Artifacts in group 4 (1.1 ± 0.4 and 1.5 ± 0.7 for the SV and SVC, respectively) were rated significantly less severe compared to group 1 (3.2 ± 1.0 and 3.0 ± 1.1), 2 (2.6 ± 1.1 and 2.3 ± 1.0) and 3 (1.9 ± 0.9 and 1.9 ± 0.7) ( p < 0.01 for all), whereas no significant difference was found between groups 1 and 2 for the subclavian vein ( p = 0.07). Attenuation in the PA was also significantly lower in group 4 (282 ± 116 HU) compared to group 1 (397 ± 137 HU), group 2 (376 ± 115 HU) and group 3 (311 ± 104 HU), but still on a diagnostic level. Conclusion Split-bolus injection provides sufficient attenuation for pulmonary DECT angiography while beam hardening artifacts arising from high density contrast material in the thoracic vessels can be reduced significantly. Keywords Dual energy CT Contrast injection protocol Perfusion analysis Pulmonary embolism 1 Introduction In order to ensure homogenous, high and consistent vascular attenuation with minimized artifacts considerable effort is being directed at optimizing injection parameters for contrast enhanced CT applications in general [1–4] and for the diagnosis of pulmonary embolism (PE) in particular [5,6] . The introduction of automated dual-syringe power injectors with the ability to inject saline solution immediately after the main contrast material bolus [6–8] has been a main development for achieving this objective. Since the introduction of Dual-Energy CT (DECT) scanners with the ability to map the distribution of iodine within the lung parenchyma, the ordinarily used contrast material protocol has major disadvantages because of the appearance of beam hardening artifacts from residual high density contrast material in the subclavian vein (VS) and the superior vena cava (SVC) [9,10] . Therefore, there is need for an examination protocol that combines both, sufficiently high enhancement in the pulmonary arteries and low incidence of beam hardening artifacts on iodine distribution map. Thus, the purpose of our study was to investigate three different biphasic and one triphasic contrast material injection protocol for dual energy pulmonary CT angiography in patients with suspected acute PE. Artifact burden on iodine distribution mapping and attenuation values in the pulmonary artery tree, descending aorta, sublavian vein and superior vena cava were retrospectively compared. 2 Materials and methods 2.1 Patients We retrospectively analyzed data of 120 patients who had undergone contrast enhanced multi-detector row CT angiography of the pulmonary arteries (pCTA) in dual source dual energy mode for clinically suspected acute pulmonary embolism (PE) at our institution between August 2007 and March 2009 ( Table 1 ). Over this period, our contrast material injection protocol for pulmonary CT angiography had undergone 3 upgrades. The benefits of the latest injection protocol, which were the basis for this retrospective analysis, were intuitively evident at clinical implementation, so this protocol had remained our clinical standard ever since. The ethics committee of our hospital did not see any ethical conflicts with this retrospective analysis and waived the need for informed patient consent. 2.2 Image acquisition All examinations were performed with a 64-slice dual source CT scanner (Somatom Definition, Siemens Healthcare). The system is equipped with two X-ray tubes and two corresponding detectors which are mounted in the gantry with an angular offset of 90°. One detector array (corresponding to tube A) provides a field of view (FOV) of 50 cm, while the other detector array (corresponding to tube B) is restricted to a FOV of 26 cm [11,12] . All scans were acquired in dual energy technique with a tube potential of 140 kV on tube A and 80 kV on tube B. Further, for scan start, the bolus tracking technique was used with a threshold of 100 HU in the pulmonary trunk and a start delay of 7 s. All examinations were contrast-enhanced with a non-ionic, low osmolar iodinated contrast material (CM) (iopamidol, Imeron 400, Bracco) injected at a flow rate of 4 ml/s through an arm vein. In group 1 ( n = 30), pulmonary CTA was performed using the following parameters: 2 × 32 × 0.6 mm collimation with z -flying focal spot technique, tube current of 100 mA s on tube A and 425 mA s on tube B, craniocaudal scan direction, inspiratory breath hold. In groups 2–4 ( n = 30 each), a 14 × 1.2 mm collimation for optimized cross-scatter correction was used. Scans were performed in caudocranial scan direction in expiratory breath hold. All images were reconstructed at a slice thickness of 1.5 mm and a position increment of 1 mm with a dedicated medium-soft convolution kernel (D30f). By default, three images series are reconstructed for dual energy scans: One series each for 80 kV, 140 kV, and one mixed series merging 70% of the 140 kV and 30% of the 80 kV data to generate the impression of a regular 120 kV image (so-called M_03 series). In group 1, contrast enhancement was achieved with 90 ml CM followed by a 30 ml saline chaser bolus. In group 2, 80 ml CM followed by 100 ml saline chaser bolus were used. In group 3, the contrast bolus was reduced to 60 ml with a 100 ml saline chaser bolus. In group 4, a triphasic split-bolus protocol was used, with injection of 50 ml of pure CM in the first phase, followed by a constant volume of 30 ml of a 70%:30% NaCl/CM mixture and 50 ml of pure NaCl in the third phase. The desired mixing ratio can be freely selected on the control panel of the used dual-syringe power injector (Injektron CT2, Medtron). The mixing ratio will be achieved by simultaneously moving the pistons of both, the NaCl and CM syringe at different speeds. 2.3 Image analysis One observer (EW), with 1 year of experience in reading chest CT scans who was blinded to the scan protocol performed attenuation measurements by placing circular regions of interest (ROI) on regular transverse sections on a medical workstation (Syngo Multimodality Workplace, Siemens). In each patient and for each target structure, three ROI were prescribed on three consecutive transverse sections depicting the respective target structure. For the pulmonary arteries, these measurements were performed at the level of the pulmonary artery trunk and on both sides in the artery of segment 1 and 10. Enhancement measurements of the descending aorta and superior vena cava were performed at the level of the aortic root. Measurements of the subclavial vein were performed in the middle part of the vessel. Region of interest size was adjusted to encompass the entire contrast-enhanced vessel lumina, avoiding the inclusion the vessel wall or surrounding soft tissue structures. The mean attenuation (in Hounsfield units) and the standard deviation within the regions of interest on three consecutive sections was calculated for each target structure. Two observers (RWB and JMK with 3 and 5 years of experience reading cardiovascular CT scans, respectively) who were blinded to the scan and injection technique reviewed in consensus each scan in random order by using the same workstation equipped with regular commercially available dual energy post processing software (Syngo 2008 G, Siemens, Forchheim, Germany). 3D color-coded iodine distribution maps representing steady-state lung perfusion were calculated based on a three-material-decomposition algorithm of soft tissue, air and iodine as described previously [13] . Using a five point scale (described below), the observers subjectively rated the occurrence and severity of steak artifacts on those perfusion maps with special regards to artifacts arising from dense contrast material in the subclavian vein as well as in the superior vena cava. Artifact severity was rated as follows: 1 = fully diagnostic iodine distribution map with no artifacts; 2 = mild streaking, but still diagnostic; 3 = moderate streaking partially obscuring pulmonary anatomy; 4 = severe streak artifacts obscuring most of the upper lung anatomy; 5 = more than 50% of the lung parenchyma non-diagnostic. In addition, the two observers compared vascular enhancement within the pulmonary artery tree between the 80 kV and the virtual 120 kV images series in every patient using a five-point scale: 1 = very weak enhancement, diagnosis of PE not possible, 2 = weak enhancement with impaired diagnostic confidence beyond the lobular level, 3 = satisfying enhancement, diagnosis of PE reliably possible to the segmental level, 4 = good enhancement, fully diagnostic to the subsegmental level, 5 = excellent enhancement with best diagnostic confidence to very peripheral arterial branches. 2.4 Statistical analysis Data analysis was performed computer-based with dedicated software (BiAS 8.4, epsilon Verlag, Frankfurt, Germany). Values for vascular attenuation and artifact rating are displayed as mean and standard deviation. Multiple comparisons of attenuation levels and artifact severity were performed with the Kruskal–Wallis test with Bonferroni–Holm correction. A p -value of <0.05 indicated statistically significant differences. 3 Results All 120 CT examinations were conducted without any problems or side effects from contrast material injection. Pulmonary CT angiography was diagnostic in all patients. Pulmonary embolism was detected in 15 patients (5 in group 1, 2 in group 2, 3 in group 3 and 5 in group 4). Enhancement in the pulmonary trunk as well as in the segmental arteries was highest in group 1 were the largest amount of contrast material was injected. There was statistically significant higher enhancement in the S1 and S10 artery for group 1 compared to all other groups ( p < 0.045). However, no significant difference was observed between groups 2, 3 and 4. No significant differences were found between the groups concerning enhancement of the descending aorta ( p > 0.6). On subjective image quality analysis, the level of pulmonary arterial enhancement on the virtual 120 kV images was rated significantly higher in group 1 where to most CM was injected compared to all other groups ( p < 0.03). On the 80 kV images, no significant difference in the level of enhancement was found between the groups ( p > 0.16) and scores where higher for all groups compared to the virtual 120 kV images (group 1: 4.8 ± 0.5 vs. 4.6 ± 0.7; group 2: 4.6 ± 0.6 vs. 4.0 ± 0.7; group 3: 4.6 ± 0.6 vs. 4.0 ± 0.8; group 4: 4.6 ± 0.6 vs. 4.1 ± 0.7). Region of interest measurements showed a decrease of attenuation in the subclavian vein from group 1 to group 4 with a statistically significant difference ( p < 0.026) except between groups 1 and 2 ( p = 0.27) and between groups 3 and 4 ( p = 0.22). Likewise, the same observation was made for the superior vena cava (statistically significant difference of CT density values with a p -value of <0.014 except between groups 1 and 2 with a p -value of 0.51). Mean artifact score for artifacts arising from the subclavian vein was highest (3.2 ± 1.0) in group 1 and gradually improved significantly (except between groups 1 and 2) in group 2 (2.6 ± 1.0), group 3 (1.9 ± 0.8) and group 4 (1.1 ± 0.3) ( Fig. 1 ). Likewise, artifact score arising from dense contrast material in the superior vena cava improved gradually from group 1 (3.0 ± 1.1), to group 2 (2.3 ± 0.9), group 3 (1.9 ± 0.7) and group 4 (1.5 ± 0.7) (no statistically significant difference between groups 2 and 3 as well as groups 3 and 4) ( Fig. 2 ). In group 1, iodine distribution analysis was severely impaired in two cases and was rated non-diagnostic (grade 5 artifacts). Tables 2 and 3 give a detailed overview on density values, artifact scores and levels of significance. 4 Discussion The results of our study suggest that a triphasic injection protocol as investigated here may improve visualization of the dual energy lung perfusion mapping by suppressing steak artifacts from inflowing contrast material and maintaining high vascular attenuation in the pulmonary arteries. High, uniform and consistent vascular attenuation within the vessel lumen is desirable for pulmonary CT angiography. Adequate attenuation is a requirement for the evaluation of the luminal integrity of the main and subsegmental pulmonary arteries and the use of two- and three-dimensional postprocessing techniques [9,14,15] . As CT of the pulmonary arteries is currently the suitable test to rule out PE, the optimization of intravenous contrast material injection protocols for appropriate attenuation of the pulmonary arteries becomes a topic of intense investigation [5,6,16–18] . The introduction of dual-syringe injectors facilitated the approach of “chasing” the main contrast medium bolus with saline [6–8,19] , which had been required careful techniques for layering contrast medium and saline in the same syringe in the era of single-barrel injectors. Flushing with saline solution only avoids pooling of contrast material in the injection system and in the arm veins, leading to better contrast material utilization [8] and improved bolus shaping, with more consistent and homogeneous attenuation of target vessels. However, with modern power injector systems, minimized contrast material volumes and difficult scan timing techniques [19] the saline chaser approach has become the clinical standard for CT of the pulmonary arteries [15,20,21] . Although this saline chaser approach is suitable for CT of the pulmonary arteries, with the advent of DECT of the lung, this approach causes beam hardening artifacts from residual dense contrast material in the superior vena cava, right heart and the subclavian vein [9,10,22] . These considerations encouraged us to shift our clinical injection protocols from a biphasic protocol, which had served us well for the evaluation of the pulmonary arteries but caused problems in the evaluation of dual energy CT lung perfusion mapping, to the described triphasic protocol. Although the triphasic protocol may appear complicated in theory, in practice, it can be stored on the injector device and selected from the menu of stored protocols. The results of our study suggest that the adoption of a triphasic injection protocols for dual energy CT angiography of the pulmonary arteries preserves the desired attenuation of the pulmonary arteries and for the dual energy color mapping of the lung parenchyma and effectively suppresses beam hardening artifacts. Use of a similar triphasic injection protocol has recently shown to be beneficial for general thoracic and aortic imaging as well as for imaging of the right heart [23,24] . Although we used unselected, consecutive patient cohorts to analyze attenuation patterns during the evaluation of our clinical injection protocols, our study was limited by its retrospective nature. For this analysis, we were concerned only with the effect of different contrast medium injection protocols on the evaluation of dual energy lung perfusion mapping, therefore, we did not evaluate the actual diagnostic performance for detection of pulmonary embolism. Although the empirically determined mixing ratio of 30% contrast medium to 70% saline for the second phase of injection works well in our practice and delivers the desired clinical results, as shown by the results of our analysis, we did not systematically investigate other possible parameter constellations and whether they would yield even better results. Last, although we have shown that our triphasic protocol improves visualization of the dual energy perfusion color maps by suppressing beam hardening artifacts while maintaining the benefits of the saline chaser technique in terms of visualization of the pulmonary arteries, this protocol still represents a standardized approach. While this works well for clinical practice, future research ought to be directed at individualized, patient based injection protocols to ensure that the minimum amount of contrast material is injected for achieving the desired contrast material attenuation throughout the target anatomic structures and DECT iodine mapping [1,2] . In conclusion, the triphasic injection as described here provides sufficient enhancement for the visualization of the pulmonary arteries and the dual energy CT lung perfusion color map, while streak artifacts from high-attenuation contrast material can generally be avoided. References [1] K.T. Bae J.P. Heiken J.A. Brink Aortic and hepatic peak enhancement at CT: effect of contrast medium injection rate—pharmacokinetic analysis and experimental porcine model Radiology 206 2 1998 455 464 [2] D. Fleischmann G.D. Rubin A.A. Bankier K. Hittmair Improved uniformity of aortic enhancement with customized contrast medium injection protocols at CT angiography Radiology 214 2 2000 363 371 [3] Y.H. Choe L.H. Phyun B.K. Han Biphasic and discontinuous injection of contrast material for thin-section helical ct angiography of the whole aorta and iliac arteries AJR Am J Roentgenol 176 2 2001 454 456 [4] K. Awai K. Hiraishi S. Hori Effect of contrast material injection duration and rate on aortic peak time and peak enhancement at dynamic CT involving injection protocol with dose tailored to patient weight Radiology 230 1 2004 142 150 [5] D.F. Yankelevitz D. Shaham A. Shah J. Rademacker C.I. Henschke Optimization of contrast delivery for pulmonary CT angiography Clin Imaging 22 6 1998 398 403 [6] K.D. Hopper T.J. Mosher C.J. Kasales T.R. TenHave D.A. Tully J.S. Weaver Thoracic spiral CT: delivery of contrast material pushed with injectable saline solution in a power injector Radiology 205 1 1997 269 271 [7] P. Haage T. Schmitz-Rode D. Hubner W. Piroth R.W. Gunther Reduction of contrast material dose and artifacts by a saline flush using a double power injector in helical CT of the thorax AJR Am J Roentgenol 174 4 2000 1049 1053 [8] H. Schoellnast M. Tillich H.A. Deutschmann Improvement of parenchymal and vascular enhancement using saline flush and power injection for multiple-detector-row abdominal CT Eur Radiol 14 4 2004 659 664 [9] C. Fink T.R. Johnson H.J. Michaely Dual-energy CT angiography of the lung in patients with suspected pulmonary embolism: initial results Rofo 180 10 2008 879 883 [10] S.F. Thieme C.R. Becker M. Hacker K. Nikolaou M.F. Reiser T.R. Johnson Dual energy CT for the assessment of lung perfusion—correlation to scintigraphy Eur J Radiol 68 3 2008 369 374 [11] T.G. Flohr C.H. McCollough H. Bruder First performance evaluation of a dual-source CT (DSCT) system Eur Radiol 16 2 2006 256 268 [12] C.H. McCollough A.N. Primak O. Saba Dose performance of a 64-channel dual-source CT scanner Radiology 243 3 2007 775 784 [13] T.R. Johnson B. Krauss M. Sedlmair Material differentiation by dual energy CT: initial experience Eur Radiol 17 6 2007 1510 1517 [14] E. Coche S. Pawlak S. Dechambre B. Maldague Peripheral pulmonary arteries: identification at multi-slice spiral CT with 3D reconstruction Eur Radiol 13 4 2003 815 822 [15] U.J. Schoepf N. Holzknecht T.K. Helmberger Subsegmental pulmonary emboli: improved detection with thin-collimation multi-detector row spiral CT Radiology 222 2 2002 483 490 [16] K.T. Bae H.Q. Tran J.P. Heiken Multiphasic injection method for uniform prolonged vascular enhancement at CT angiography: pharmacokinetic analysis and experimental porcine model Radiology 216 3 2000 872 880 [17] M. Remy-Jardin J. Remy D. Artaud F. Deschildre A. Duhamel Peripheral pulmonary arteries: optimization of the spiral CT acquisition protocol Radiology 204 1 1997 157 163 [18] I.J. Hartmann R.T. Lo J. Bakker W. de Monye P.F. van Waes P.M. Pattynama Optimal scan delay in spiral CT for the diagnosis of acute pulmonary embolism J Comput Assist Tomogr 26 1 2002 21 25 [19] M.A. Auler T. Heagy L. Aganovic R. Brothers P. Costello U.J. Schoepf Saline chasing technique with dual-syringe injector systems for multi-detector row computed tomographic angiography: rationale, indications, and protocols Curr Probl Diagn Radiol 35 1 2006 1 11 [20] M. Remy-Jardin M. Pistolesi L.R. Goodman Management of suspected acute pulmonary embolism in the era of CT angiography: a statement from the Fleischner Society Radiology 245 2 2007 315 329 [21] U.J. Schoepf P. Costello CT angiography for diagnosis of pulmonary embolism: state of the art Radiology 230 2 2004 329 337 [22] F. Pontana J.B. Faivre M. Remy-Jardin Lung perfusion with dual-energy multidetector-row CT (MDCT): feasibility for the evaluation of acute pulmonary embolism in 117 consecutive patients Acad Radiol 15 12 2008 1494 1504 [23] N. Funabashi H. Sato M. Asano T. Nakayama I. Komuro Utility of triple channel injection of contrast material with mixture of saline, with acquisition in the cephalic direction for arterial trees in the thorax using multislice computed tomography Int J Cardiol 113 1 2006 97 103 [24] J.M. Kerl J.G. Ravenel S.A. Nguyen Right heart: split-bolus injection of diluted contrast medium for visualization at coronary CT angiography Radiology 247 2 2008 356 364
MoreTranslated text
Key words
Dual energy CT,Contrast injection protocol,Perfusion analysis,Pulmonary embolism
AI Read Science
Must-Reading Tree
Example
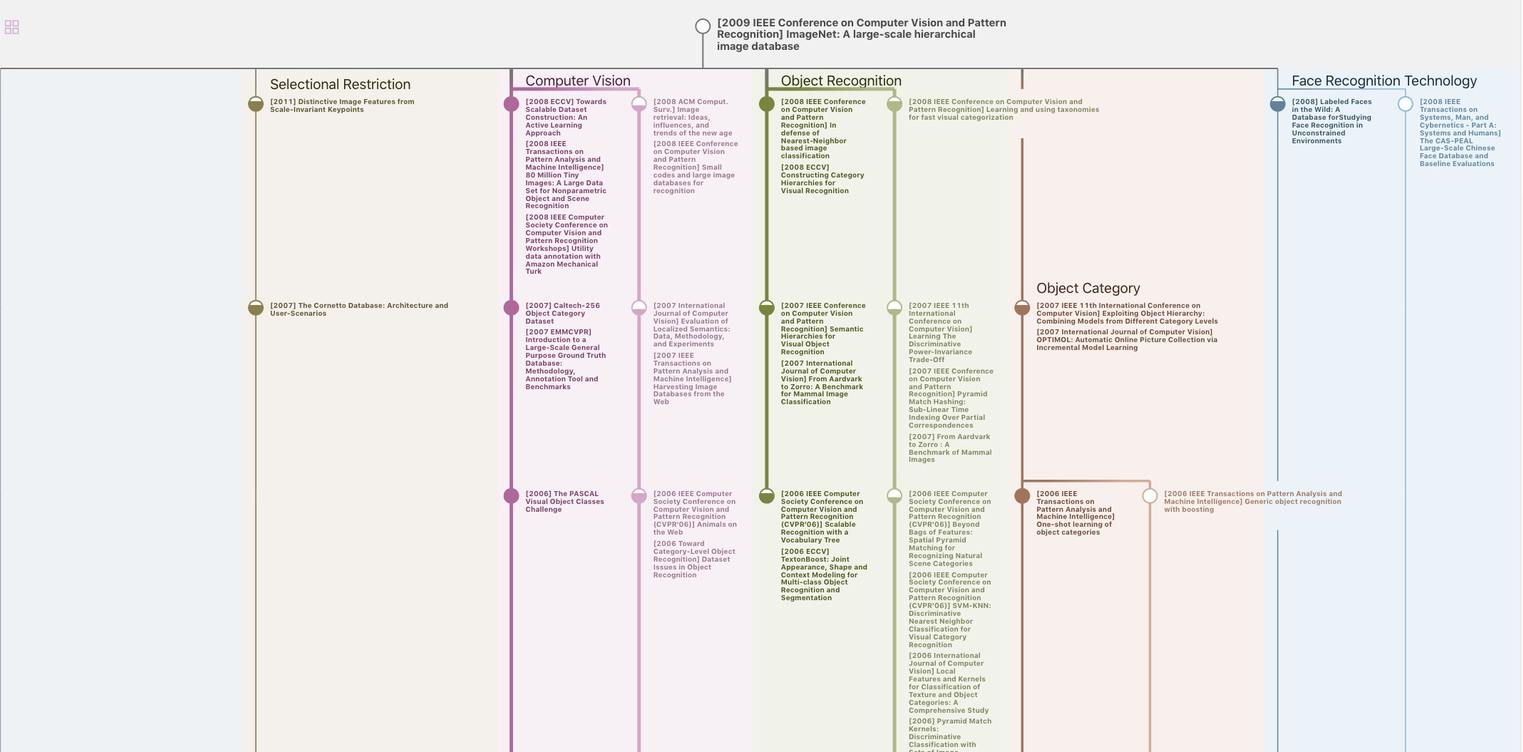
Generate MRT to find the research sequence of this paper
Chat Paper
Summary is being generated by the instructions you defined