Commutability of NIST SRM 1955 Homocysteine and Folate in Frozen Human Serum with selected total homocysteine immunoassays and enzymatic assays
Clinica Chimica Acta(2008)
摘要
Results Normalized residuals ranged from − 2.65 to 2.19 for SRM 1955. The median interlaboratory/interassay imprecision (CV) was ≈ 4% for patient specimens and ranged from ≈ 3% to ≈ 7% for SRM 1955. The median intra-assay imprecision ranged from ≈ 1% to ≈ 13%. Orthogonal residuals, as a descriptor of assay accuracy, ranged from 0.29 to 7.71 and from 0.20 to 2.22 for patient specimens and SRM 1955 samples, respectively. Conclusion The current study suggests that SRM 1955 is commutable with the investigated tHcy assays; however, a broader specimen set needs to be evaluated to completely substantiate this conclusion. Abbreviations tHcy total homocysteine CVD cardiovascular disease FPIA fluorescence polarization immunoassay CIA chemiluminescence immunoassay Keywords Cardiovascular disease Commutability Homocysteine Immunoassay Enzymatic assay Standard Reference Material 1 Introduction Hyperhomocysteinemia is considered a graded, independent risk factor for cardiovascular disease (CVD) in the general population [1] , but it is not known with absolute certainty whether elevated plasma homocysteine (Hcy) levels cause CVD or whether elevated plasma Hcy levels are a direct consequence of CVD [2] . Accurate assessment of Hcy levels is complicated by the presence and instability of a variety of reduced (free Hcy) and oxidized (homocysteine, Hcy thiolactone, etc.) Hcy forms in the body. Because of this, the quantitative determination of Hcy has generally been accomplished via the assessment of “total homocysteine (tHcy)” which precludes the need to measure multiple, unstable Hcy forms. The normal adult tHcy reference range is not well defined, but is usually reported as ≈ 5 μmol/l to ≈ 15 μmol/l [3–5] . Since the vascular pathology of Hcy was initially recognized by McCully [6] , many different approaches to tHcy measurement, ranging from radioenzymic assay [7] to liquid chromatography/tandem mass spectrometry (LC/MS/MS) [8] , have been developed. Current approaches to plasma tHcy measurement are based predominantly upon chromatographic assays, enzymatic assays or immunoassays, and in general, the measured tHcy levels are concordant among the different assay formats [9] . However, the intralaboratory and interlaboratory variability (imprecision) of the measurements are often quite poor [9–11] . Typical coefficients of variation (CVs) for plasma tHcy measurements range from 3% to 24% (intralaboratory) and from 6% to 16% (interlaboratory) [9–11] . These CVs correspond to plasma samples with tHcy levels ranging from ~ 5 μmol/l to ~ 50 μmol/l. In order to reduce laboratory measurement variability and improve the accuracy and reliability of tHcy measurements overall, the Hcy research community has requested the development of reference methods and reference materials [3,4,10,11] . The National Institute of Standards and Technology (NIST), in collaboration with the Centers for Disease Control and Prevention (CDC), has developed a Standard Reference Material (SRM 1955 Homocysteine and Folate in Frozen Human Serum), in part, to address the aforementioned tHcy measurement needs [3,4,10–14] . SRM 1955 has been assigned certified values for tHcy and 5-methyl-tetrahydrofolic acid (5MT) [12] . Higher-order, candidate reference methods, based on stable isotope-dilution LC/MS/MS, were utilized to assign certified values to tHcy and 5MT [15,16] . SRM 1955 is intended primarily for use in evaluating the performance and accuracy of tHcy clinical assays, and as such, there exists a requirement to experimentally demonstrate that the reference material exhibits the same intermethod behavior as patient specimens for a given pair of test methods. In other words, as originally proposed by Rej et al. [17–20] , all new clinical reference materials ideally should be assessed for commutability before being adopted for general use by the clinical community. The present report describes a preliminary interlaboratory assessment of the commutability of NIST SRM 1955 Levels 1, 2 and 3 with selected commercially available tHcy immunoassays and enzymatic assays. The study was designed to provide commutability information for SRM 1955; it was not designed to rigorously assess the accuracy and imprecision of the measurement procedures. Nevertheless, the report does provide limited accuracy and imprecision data for 14 of the investigated assays. 2 Materials and methods 2.1 Participating laboratories The participating laboratories (a total of 14) and associated tHcy assays are listed in Table 1 . We attempted to include 2 or more independent laboratories for each assay principle/platform designation. For example, Lab code A1 and A2 were two independent laboratories (measuring tHcy via FPIA) that both utilized identical tHcy assay platforms (platform A). Deviations from the intended study scheme occurred for Lab code F1 and Lab codes G1, G2, G3 and G4. Lab code F1 was the singular study representative for this recently marketed enzymatic assay [28] . Because the assays performed by Lab codes G1 and G4 utilized the same platform, the same assay kit format and independent sets of test specimens, we compared these two Lab codes in order to confirm the commutability of SRM 1955 within the G assay series. 2.2 Study protocol and specimens At this time, there exists no specific recommendation or approved guideline for conducting commutability studies on reference materials or analyzing the resulting data. However, the current approved guideline for the evaluation of matrix effects (EP14-A2) provides some suggestions for designing a commutability study with various options for statistical analysis of the data [30] . The present study was loosely modeled after the suggested protocols in EP14-A2 with additional refinements to the data analysis approach as deemed necessary. Participants were asked to measure tHcy levels in a total of 69 serum samples using their laboratory's tHcy assay procedure and platform. They were instructed to utilize their calibrants, standards, quality control samples and blanks for the study. Specifically, each participant was provided with 3 sets of 20 normal-donor serum samples (patient specimens, 500 μl/vial) and 3 sets of NIST SRM 1955 — Level 1, 2, 3 (processed samples, 1000 μl/vial) [30] . All patient specimens were putatively normal, randomly selected single-donor serum pools obtained from Tennessee Blood Bank (Memphis, TN), while the processed samples were prepared from a multiple-donor serum pool as previously described [14] . All patient specimens and processed samples were screened for tHcy levels by liquid chromatography coupled with fluorescence detection (LC/FD) [31] before beginning the study. Specimens from patients with known hyperhomocysteinemia or other disease conditions resulting in hyperhomocysteinemia were not included in this initial study. Participants were instructed to perform 1 assay on each patient specimen and processed sample on each of 3 days using fresh vials on each day. The commutability study was initiated in August of 2006 and completed by November of 2006. 2.3 Comparative assay The study utilized a tHcy comparative assay (candidate reference assay) based on analyte extraction procedures and isotope-dilution LC/MS/MS quantitation conditions previously published and applied to the assignment of certified tHcy levels in SRM 1955 [15] . 2.4 Data analysis For the assessment of SRM 1955 commutability, an error-in-variables (EIV) statistical model [32,33] based closely on the Deming regression technique [34] was applied to each of the 16 evaluated assay data sets. Variants of the EIV model have been recently proposed for and/or successfully applied to the assessment of the commutability of control materials [30,35,36] . For the EIV model, a weighted linear least-squares regression analysis that took into account the uncertainties associated with both the x -axis and y -axis [37] was utilized to compare patient specimen data points from each evaluated assay against the corresponding patient specimen data points from the comparative assay. Each evaluated assay (Lab codes A1 through G4 in Table 1 ) was assigned to the y -axis and the comparative LC/MS/MS assay was utilized as the reference assay ( x -axis). A regression equation was obtained from the patient specimen data for each evaluated assay-comparative assay pair; the resultant slope and intercept values were then used to calculate the predicted y values ( ŷ ) for each sample x value: (1) y ˆ = ( s l o p e ⋅ x ) + i n t e r c e p t The residuals along the y -axis were calculated as the difference between the y value and the predicted value ( ŷ ). Normalized residuals were then calculated by dividing the residuals ( y − ŷ ) by the standard deviation (SD) of the residuals from the patient specimen data (for a given evaluated assay). For each level of SRM 1955, commutability was determined by assessing whether its normalized residual was within an interval of ± 3.0. SRM 1955 samples that resulted in normalized residuals > ± 3.0 were deemed noncommutable with the given evaluated assay. As a supplement to the normalized residual approach, the calculated 2-tailed 95% prediction interval for the patient specimen data about the resultant EIV regression lines for each evaluated assay were calculated as defined by EP14-A2.The procedures used for assessing assay imprecision and accuracy can be found in the Supplementary data to the manuscript. 3 Results 3.1 Commutability Patient specimen and SRM 1955 tHcy data generated with each of the 14 evaluated assays were independently plotted against patient specimen and SRM 1955 tHcy data generated with the comparative assay. The EIV regression line through the patient specimen data and the corresponding two-tailed 95% prediction interval [30] were calculated and plotted for each evaluated assay. Additionally, the EIV regression line through the patient specimens was extrapolated to points below SRM Level 1 and to points above SRM Level 3. For illustrative purposes, all plots show the data point for the highest concentration patient specimen (≈ 31 μmol/l tHcy), but this point was excluded from the regression because it produced a bias in the final regression line (see rationale below). Representative plots, such as those depicted in Fig. 1 for immunoassays A1 and A2 ( Table 1 ), show that the data points for SRM 1955 Level 2 lie within the 95% prediction interval of the EIV regression lines generated from the 19 patient specimens. The data points (inclusive of both the x and y uncertainties) for SRM 1955 Levels 1 and 3 lie on or near the extrapolated EIV regression lines for assays A1 and A2 but outside of the range defined by the 95% prediction interval. Fig. 2 shows representative plots from the evaluation of enzymatic assays G1 and G4 ( Table 1 ). The behavior of SRM 1955 with assays G1 and G4 is quite similar to the behavior observed with assays A1 and A2. The calculated prediction intervals for the G series assays are visually broader compared to the A series assays which reflects the increased scatter in the G series assay data. The observations noted for the A and G series assays are characteristic of the tHcy assays evaluated in this study (see Figs. S1–S5 in the data supplement). Modified bias plots [19] showing all of the normalized residuals from the EIV statistical assessment of SRM 1955 commutability are shown in Fig. 3 . Examination of the normalized residuals with respect to tHcy levels from all of the patient specimens ( Fig. 3 a) illustrates an abnormal distribution of the residuals with a significant bias towards the high-concentration patient specimen. This residual distribution suggests that the high-concentration patient specimen is responding within the assays in a manner that is fundamentally different from the other patient specimens with lower tHcy levels. Close inspection of Fig. 3 a shows that all of the evaluated assays generate normalized residuals within the ± 3.0 interval for SRM 1955 Levels 1, 2 and 3. In order to eliminate the bias that was introduced into the normalized residuals data set by the high-concentration patient specimen, the specimen was excluded from the data set and the modified bias plot was regenerated ( Fig. 3 b). The resulting patient specimen residuals tended towards a more normal distribution. Inspection of Fig. 3 b shows that all of the evaluated assays continue to generate normalized residuals within the ± 3.0 interval for SRM 1955 Levels 1, 2 and 3. The tabulated SRM 1955 normalized residual data for all of the evaluated assays (based on the non-biased regression analysis) are presented in Table S1 in the data supplement. 3.2 Imprecision Results from the assessment of interlaboratory, interassay and intra-assay variability are presented in Table 2 . The median interlaboratory and interassay CV was < 4% for the patient specimens and ≤ 7% for each level of SRM 1955. The median interlaboratory and interassay CVs are inversely correlated with the concentration of tHcy in the SRM 1955 samples. Regarding intra-assay variability, the CIA assay (assays D1 and D2) exhibited the largest median CV (≈ 11%), while the FPIA assay (assays A1 and A2) exhibited the smallest median CV (≈ 2%) for the patient specimens. The CIA assay (assays D1 and D2 exhibited the largest median CV for SRM 1955 Level 1 (≈ 13%) and Level 2 (≈ 10%); however, the ELISA assay (assays E1 and E2) exhibited the largest median CV for SRM 1955 Level 3 (≈ 7%). The FPIA assay (assays A1 and A2) exhibited the smallest median CV (≤ 3%) for each level of SRM 1955. The imprecision results for all SRM samples and patient specimens are presented in Tables S2a and S2b in the data supplement. 3.3 Accuracy Results from the assessment of assay accuracy are presented in Table 3 in terms of orthogonal residuals calculated relative to the identity lines drawn in Figs. 1 and 2 and in Figs. S1–S5 (data supplement). The evaluated assays were assessed on the basis of the presence (large orthogonal residual) or absence (small orthogonal residual) of bias in relation to the comparative assay. In terms of the 19 patient specimens within the normal adult tHcy reference range, the assays exhibiting the least bias were the A series and F1 assays. The assays exhibiting the most bias were the D and E series assays. In terms of the high-concentration patient specimen, the assays exhibiting the least bias were the B and D series assays. The assays exhibiting the most bias were the C series and F1 assays. In terms of the SRM 1955 samples, the assays exhibiting the least bias were the A and G series assays. The assays exhibiting the most bias were the B and D series assays. 4 Discussion SRM 1955 was specifically designed as a primary control material for serum-based tHcy clinical assays, however, each concentration level of SRM 1955 has slightly different analytical specifications [12,14] . The base serum pool, containing only endogenous Hcy forms, was developed into Level 2 of SRM 1955. Level 1 of SRM 1955 was prepared by diluting the base serum pool 1:1 (volume fractions) with standardized phosphate-buffered saline. Level 3 of SRM 1955 was prepared by spiking a known amount of l -homocysteine primary standard into the base serum pool. Thus, in comparison to Level 2 of SRM 1955, Level 1 of SRM 1955 has a reduced overall protein concentration and Level 3 of SRM 1955 contains both natural and synthetic forms of l -homocysteine. Previous researchers have noted that candidate control materials that have been processed (e.g. by dilution with buffers or by spiking in synthetic analytes) often behave differently from patient specimens in certain assay systems [18,19] . The subtle differences in the matrices for each level of SRM 1955 coupled with the fact that SRM 1955 represents a multi-donor serum pool, made it necessary to ascertain the commutability of SRM 1955 within the framework of the current clinical tHcy assays. Regression through all 20 of the patient specimen data points for each evaluated assay-comparative assay pairing ( Figs. 1, 2 and Figs. S1–S5 in the data supplement) results in a distinct, positive bias for the high-concentration patient specimen within the data set. The overall study mean (30.5 μmol/l tHcy) for this patient specimen was approximately 7% higher than the comparative assay mean (28.5 μmol/l tHcy), even though the means are concordant within the calculated measurement uncertainties. Patient specimen data points were previously verified during the initial LC/FD screening of the study samples; the LC/FD mean values were in 95% agreement with the LC/MS/MS values (inclusive of the high-concentration patient specimen). Hence, there did not appear to be any technical or assay-related problem associated with the analysis of the high-concentration patient specimen. A more detailed investigation into the anomalous behavior of the high-concentration patient specimen with the immunoassays/enzymatic assays is warranted; a study incorporating a substantial number of high-concentration patient specimens would help characterize the source of the apparent bias. Exclusion of the high-concentration patient specimen eliminated the positive bias in the regression, but still allowed for reasonable extrapolation of the EIV regression lines. Further, the remaining set of 19 patient specimens (6.0 μmol/l tHcy to 15.2 μmol/l tHcy) was more representative of the certified tHcy concentrations covered by SRM 1955 (4.0 μmol/l tHcy to 18.0 μmol/l tHcy) and also of the normal adult tHcy reference range (5.0 μmol/l tHcy to 15.0 μmol/l tHcy). Inspection of the plots specifically showed that the data points for SRM Level 2 lay on/near the regression lines for all of the evaluated assays. These observations provided strong evidence suggesting that SRM Level 2 was commutable with patient specimens within the normal adult reference range, for each of the evaluated assays. Further inspection of the plots showed that the data points for SRM Levels 1 and 3 did not specifically lie on/near the regression lines, but the data points did lie on/near the “extrapolated” regression lines. These observations provided some evidence to suggest that SRM Levels 1 and 3 were commutable with patient specimens for each of the evaluated assays, but the evidence was not nearly as convincing as it was for SRM Level 2. The two-tailed 95% prediction intervals for the patient specimens in Figs. 1, 2 and Figs. S1–S5 in the data supplement are provided for illustrative purposes only. Application of the prediction intervals for the assessment of commutability is restricted to SRM 1955 Level 2 (≈ 10 μmol/l tHcy), because Level 2 is sufficiently bracketed within the range of patient specimen data. However, visual extrapolation of the prediction intervals along the regression lines for all of the evaluated assays further suggested that SRM 1955 Levels 1 and 3 may also be commutable with each of the assays. To more critically investigate the commutability of SRM 1955 with the evaluated assays, modified bias plots of the normalized residuals based on all of the collected tHcy data were constructed. Fig. 3 graphically demonstrates the commutable behavior of SRM 1955 Level 1, 2 and 3 with all of the evaluated assays, both in the presence ( Fig. 3 a) and absence ( Fig. 3 b) of the high-concentration patient specimen. Each of the evaluated assays returned normalized residuals within the ± 3.0 boundary (range ≈ − 2.7 to ≈ 2.2) for SRM 1955 Levels 1, 2 and 3. Thus, the data resulting from the modified bias plots ( Fig. 3 ) and from the normalized residual determinations ( Table S1 ), further suggests that SRM 1955 Levels 1, 2 and 3 may be commutable with patient samples for all of the evaluated assays. However, because the preliminary patient specimen set utilized for this study, did not fully bracket SRM 1955 Level 1 and SRM 1955 Level 3, absolutely conclusive statements regarding commutability of SRM 1955 are not warranted (see full explanation below). In the study, the ± 3.0 boundary for the normalized residuals was utilized based on its successful application in previous commutability studies [17,35] . Further, the Gaussian distribution of the normalized residuals from the SRM 1955 samples presented no compelling reason to constrict or expand the ± 3.0 boundary and the boundary was deemed appropriate for the demonstrated imprecision (intra-assay CV, see below) of the evaluated assays. However, it is recognized that there might exist situations where it would be desirable to use more restrictive boundary criteria for the establishment of reference material commutability [17] . For those situations, it is important to establish that the imprecision of the clinical assays are within an acceptable limit. There were no apparent connections between the imprecision assessments ( Table 2 ) and the commutability evaluations ( Table S1 and Fig. 3 ) for the 14 evaluated assays. However, it should be noted that some assays had wider prediction intervals (increased measurement scatter) than other assays (compare Figs. 1 and 2 ) and this increased measurement scatter could have had an impact on the overall commutability evaluation. The average interlaboratory CV for the serum-based patient specimens in the present study was ≈ 5%. This contrasts with the average interlaboratory CV (among laboratory CV) of ≈ 9% [11,38] previously reported for the determination of tHcy in plasma-based samples using predominantly chromatographic assays. The improvement in the interlaboratory precision could possibly be due to the more complete automation of most immunoassays and enzymatic assays in comparison to the partially-automated chromatographic measurement procedures. There were no significant differences between the average interlaboratory and interassay CVs for any of the patient specimens or SRM 1955 samples. Overall, the best performing assay in terms of intra-assay imprecision was the FPIA assay on the Abbott IMx platform (assays A1 and A2); for all samples (patient specimens and SRM 1955 samples combined) the median intra-assay CV was ≤ 3%. Other researchers have obtained similar or slightly higher CVs (3–5%) for the FPIA assay using an identical platform [11] . The FPIA assay on the Abbott AxSym platform (assays B1 and B2) also performed well in terms of imprecision; all analyses resulted in median intra-assay CVs < 3%, except for the analyses of SRM 1955 Level 1 which resulted in median CVs of approximately 7%. Except for the increased CV exhibited for SRM 1955 Level 1, the imprecision of the FPIA assay on the AxSym platform is similar to the imprecision (CVs = 4% to 5%) recently reported by Lonati et al. [22] . The median intra-assay imprecision of the CIA assay on the Bayer Advia Centaur platform (assays C1, C2 and C3) was ≤ 4% for all samples which is in excellent concordance with the published intra-assay imprecision values (CVs = 2% to 4%) [24] . On the other hand, the imprecision data for the CIA assay on the DPC Immulite 2000 platform (assays D1 and D2) were not, in general, concordant with published literature data. The CIA assay on the DPC Immulite platform exhibited median intra-assay imprecision values (CVs) for all samples ≥ 10%, except for SRM 1955 Level 3, which returned a median intra-assay CV of 3%. Published imprecision values (CVs = 5% to 8%) for the CIA assay [25] are generally better than those obtained in the present study. The semi-automated Axis-Shield ELISA assay (assays E1 and E2) exhibited median intra-assay imprecision CVs ≥ 7% for all samples. The intra-assay imprecision data for the ELISA assay are concordant with published imprecision data (CVs = 5% to 8%) [26,27] . The Catch enzymatic assay (assays G1 and G4) performed well in terms of imprecision; all analyses resulted in median intra-assay CVs < 5%. Published imprecision values (CVs = 2% to 3%) for the Catch assay [29] are slightly better than those obtained in the present study. Additionally, we found no obvious connections between the accuracy assessments ( Table 3 ) and the commutability evaluations ( Table S1 and Fig. 3 ) for the 14 evaluated assays. A general accounting of the orthogonal residuals across the 19 patient specimens and the combined SRM 1955 samples indicates that the FPIA assay (assays A1 and A2) exhibits the lowest level of bias and the CIA assay (assays D1 and D2) exhibits the highest level of bias for the determination of tHcy. In this preliminary study, the collected data suggested that SRM 1955 Levels 1, 2 and 3 was commutable with patient specimens within the normal adult reference range, for the evaluated tHcy immunoassay and enzymatic assay measurement procedures. Hence, SRM 1955 could potentially serve as a useful control or calibrator material for these assays. However, due to the lack of a statistically significant number of patient specimens in the study with nominal tHcy concentrations below the certified tHcy concentration in SRM 1955 Level 1 and/or above the certified tHcy concentration in SRM 1955 Level 3, the reported commutability conclusions for the evaluated assays are tentative and are only valid between the concentration extremes encompassed by SRM 1955 Levels 1 and 3. It must be emphasized that the commutability assessment for Levels 1 and 3 were based on extrapolated patient specimen data and it can not be known for certain how “true” patient specimen data would have influenced the regression lines. We made the assumption that the patient specimens would behave normally both below Level 1 and above Level 3, however, the behavior of the single high-concentration patient specimen in the present study suggests that this assumption may not be completely valid. However, we have strong confidence in the commutability assessment for Level 2 because patient specimen data completely surrounded this reference material in all of the evaluated assays; no extrapolated patient specimen data was necessary for the Level 2 assessment. To positively confirm the commutability of Levels 1 and 3 with the evaluated assays, a follow-up commutability study involving patient specimens with tHcy levels both below Level 1 and above Level 3 is justified. It must be emphasized that the present commutability study was inherently limited in scope and outcome due to a combination of the design of SRM 1955 and to the lack of sufficient study specimens with tHcy concentrations ≥ 20 μmol/l. SRM 1955 Levels 1, 2 and 3 (3.98 μmol/l tHcy to 17.7 μmol/l tHcy) covers only a limited range (normal and borderline high) of possible tHcy test results and thus the reference material is clearly not useful for assessing the accuracy of test results from most clinically-important patient specimens (tHcy ≥ 20 μmol/l). It should also be noted that many of the current tHcy clinical assays are capable of achieving analytical linearities of ≥ 50 μmol/l, greatly exceeding the mean certified tHcy concentration (17.7 μmol/l) for SRM 1955 Level 3. Hence, the utility of SRM 1955 is limited to assessing the accuracy of patient results from mostly normal and borderline high patient specimens. Compounding the limitations in scope and outcome for the commutability study is the fact that only one patient specimen that had a tHcy concentration in the clinically-important realm (tHcy ≥ 20 μmol/l) was included and then ultimately excluded from analysis of the commutability data. Thus, the final statistical and commutability evaluations were based solely on data from patient specimens with normal and borderline high tHcy concentrations. The study would have been improved and more valuable if the SRM material had been designed with a wider range of certified tHcy concentrations and if the study itself had included sufficient numbers of patient specimens with tHcy concentrations ≥ 20 μmol/l. Calibration extrapolations by users of SRM 1955 beyond the prediction intervals described by the tri-level material cannot be supported nor recommended based on the current data, and would require further validation at the assay-specific level. Acknowledgements We kindly thank Michael Welch (NIST) and Jan Møller (Aarhus University Hospital, Denmark) for their helpful discussions. We graciously thank Maryanne Mildner (NIST) for her help in executing the study. We would also like to thank Zia Fazili (CDC) for help in preparing the study specimens. The following individuals are recognized and sincerely appreciated for their willingness to participate in the study: Mark Brown and Stewart Kennedy, Axis-Shield Diagnostics, Ltd., Dundee, Scotland; Prakash Tewari and Sabrinah Chapman, Siemens Medical Solutions Diagnostics, Tarrytown, NY; Vibeke Leholk, Bispebjerg Hospital, Copenhagen, Denmark; Raymond Liedtke, Catch, Inc., Bothell, WA; Nader Rifai and Gary Bradwin, Harvard University/Boston Children's Hospital, Cambridge, MA; Véronique Ducros, Centre Hospitalier Universitaire de Grenoble, Grenoble, France; Brian Gilfix and Paula Hiltz, MUHC–Royal Victoria Hospital, Montreal, Quebec, Canada; James Donnelly, NYU/Bellevue Medical Center, New York, NY; Carole Johnston and Cynthia Prendergast, Oxford University, Oxford, England; Wolfgang Herrmann and Rima Obeid, Saarland University, Homburg, Germany; Jan Møller, Aarhus University Hospital, Aarhus, Denmark; Regina Dempsey, St. James's Hospital, Dublin, Ireland; and Barbro Liljeqvist, Sundsvalls Sjukhus, Sundsvall, Sweden. Appendix A Appendix A Supplementary data Supplementary data associated with this article can be found, in the online version, at doi:10.1016/j.cca.2008.05.016 . References [1] C.J. Boushey S.A. Beresford G.S. Omenn A.G. Motulsky A quantitative assessment of plasma homocysteine as a risk factor for vascular disease. Probable benefits of increasing folic acid intakes JAMA 274 1995 1049 1057 [2] S.R. Lentz W.G. Haynes Homocysteine: is it a clinically important cardiovascular risk factor? Cleve Clin J Med 71 2004 729 734 [3] V. Ducros K. Demuth M.P. Sauvant Methods for homocysteine analysis and biological relevance of the results J Chromatogr B Analyt Technol Biomed Life Sci 781 2002 207 226 [4] H. Refsum A.D. Smith P.M. Ueland Facts and recommendations about total homocysteine determinations: an expert opinion Clin Chem 50 2004 3 32 [5] K. Rasmussen J. Moller M. Lyngbak A.M. Pedersen L. Dybkjaer Age- and gender-specific reference intervals for total homocysteine and methylmalonic acid in plasma before and after vitamin supplementation Clin Chem 42 1996 630 636 [6] K.S. McCully Vascular pathology of homocysteinemia: implications for the pathogenesis of arteriosclerosis Am J Path 56 1969 111 128 [7] H. Refsum S. Helland P.M. Ueland Radioenzymic determination of homocysteine in plasma and urine Clin Chem 31 1985 624 628 [8] M.B. Satterfield L.T. Sniegoski M.J. Welch B.C. Nelson C.M. Pfeiffer Comparison of isotope-dilution mass spectrometry methods for the determination of total homocysteine in plasma and serum Anal Chem 75 2003 4631 4638 [9] A. Tripodi V. Chantarangkul R. Lombardi A. Lecchi P.M. Mannucci M. Cattaneo Multicenter study of homocysteine measurement—performance characteristics of different methods, influence of standards on interlaboratory agreement of results Thromb Haemost 85 2001 291 295 [10] N.Q. Hanson J.H. Eckfeldt K. Schwichtenberg O. Aras M.Y. Tsai Interlaboratory variation of plasma total homocysteine measurements: results of three successive homocysteine proficiency testing surveys Clin Chem 48 2002 1539 1545 [11] C.M. Pfeiffer D.L. Huff S.J. Smith D.T. Miller E.W. Gunter Comparison of plasma total homocysteine measurements in 14 laboratories: an international study Clin Chem 45 1999 1261 1268 [12] NIST SRM 1955 Homocysteine and Folate in Frozen Human Serum — Certificate of Analysis, https://srmorsnistgov/certificates/view_cert2gifcfm?certificate=1955 . [13] M.B. Satterfield B.C. Nelson L.T. Sniegoski M.J. Welch New standard reference material for homocysteine and folate FASEB J 18 2004 A177 [14] M.B. Satterfield L.T. Sniegoski K.E. Sharpless Development of a new standard reference material: SRM 1955 (homocysteine and folate in human serum) Anal Bioanal Chem 385 2006 612 622 [15] B.C. Nelson M.B. Satterfield L.T. Sniegoski M.J. Welch Simultaneous quantification of homocysteine and folate in human serum or plasma using liquid chromatography/tandem mass spectrometry Anal Chem 77 2005 3586 3593 [16] C.M. Pfeiffer Z. Fazili L. McCoy M. Zhang E.W. Gunter Determination of folate vitamers in human serum by stable-isotope dilution tandem mass spectrometry and comparison with radioassay and microbiologic assay Clin Chem 50 2004 423 432 [17] C. Franzini Commutability of reference materials in clinical chemistry J Int Fed Clin Chem 5 1993 169 173 [18] R. Rej Accurate enzyme activity measurements Arch Pathol Lab Med 117 1993 352 364 [19] J.G. Middle J.C. Libeer V. Malakhov I. Penttila Characterisation and evaluation of external quality assessment scheme serum Clin Chem Lab Med 36 1998 119 130 [20] R. Rej R.W. Jenny J.P. Bretaudiere Quality control in clinical chemistry characterization of reference materials Talanta 31 1984 851 862 [21] M.T. Shipchandler E.G. Moore Rapid, fully automated measurement of plasma homocysteine with the Abbott IMx analyzer Clin Chem 41 1995 991 994 [22] S. Lonati C. Novembrino S. Ippolito Analytical performance and method comparison study of the total homocysteine fluorescence polarization immunoassay (FPIA) on the AxSYM analyzer Clin Chem Lab Med 42 2004 228 234 [23] P. Pernet E. Lasnier M. Vaubourdolle Evaluation of the AxSYM homocysteine assay and comparison with the IMx homocysteine assay Clin Chem 46 2000 1440 1441 [24] P.C. Tewari B. Zhang B.I. Bluestein Analytical and clinical evaluation of the Bayer ADVIA Centaur homocysteine assay Clin Chim Acta 342 2004 171 178 [25] J. Moller L. Ahola L. Abrahamsson Evaluation of the DPC Immulite 2000 assay for total homocysteine in plasma Scand J Clin Lab Invest 62 2002 369 374 [26] F. Frantzen A.L. Faaren I. Alfheim A.K. Nordhei Enzyme conversion immunoassay for determining total homocysteine in plasma or serum Clin Chem 44 1998 311 316 [27] I. Quintana D. Freeman C. Galarza A. Murua J.D. Spence L. Kordich Validation of an enzyme immunoassay for the determination of total homocysteine in plasma Blood Coagul Fibrinolysis 11 2000 235 238 [28] Axis-Shield Enzymatic Homocysteine Assay — Assay Information Document, http://wwwaxis-shieldcom/indexcfm?action=productdetail&productid=7&cattitle=Cardiovascular (Accessed January 2007). [29] M.D. Kellogg R. Parker A. Ricupero N. Rifai Evaluation of an enzymatic homocysteine assay for the Hitachi series chemistry analyzer Clin Chim Acta 354 2005 117 122 [30] F.D. Lasky M.H. Kroll D.W. Tholen Evaluation of matrix effects; approved guideline Clinical and Laboratory Standards Institute: EP14-A2 25 2005 1 29 [31] C.M. Pfeiffer D.L. Huff E.W. Gunter Rapid and accurate HPLC assay for plasma total homocysteine and cysteine in a clinical laboratory setting Clin Chem 45 1999 290 292 [32] M.J.T. Milton P.M. Harris I.M. Smith A.S. Brown B.A. Goody Implementation of a generalized least-squares method for determining calibration curves from data with general uncertainty structures Metrologia 43 2006 S291 S8 [33] D. Stockl K. Dewitte L.M. Thienpont Validity of linear regression in method comparison studies: is it limited by the statistical model or the quality of the analytical input data Clin Chem 44 1998 2340 2346 [34] R.F. Martin General Deming regression for estimating systematic bias and its confidence interval in method-comparison studies Clin Chem 46 2000 100 104 [35] R.H. Christenson S.H. Duh F.S. Apple Towards standardization of cardiac troponin I measurements part II: assessing commutability of candidate reference materials and harmonization of cardiac troponin I assays Clin Chem 52 2006 1685 1692 [36] R. Dominici E. Cabrini G. Cattozzo Intermethod variation in serum carcinoembryonic antigen (CEA) measurement: fresh serum pools and control materials compared Clin Chem Lab Med 40 2002 167 173 [37] ISO International Standard 6143:2001(E) Gas analysis - comparison methods for determining and checking the composition of calibration gas mixtures. Geneva: International Organization for Standardization. [38] J. Moller L. Christensen K. Rasmussen An external quality assessment study on the analysis of methylmalonic acid and total homocysteine in plasma Scand J Clin Lab Invest 57 1997 613 619
更多查看译文
关键词
tHcy,CVD,FPIA,CIA
AI 理解论文
溯源树
样例
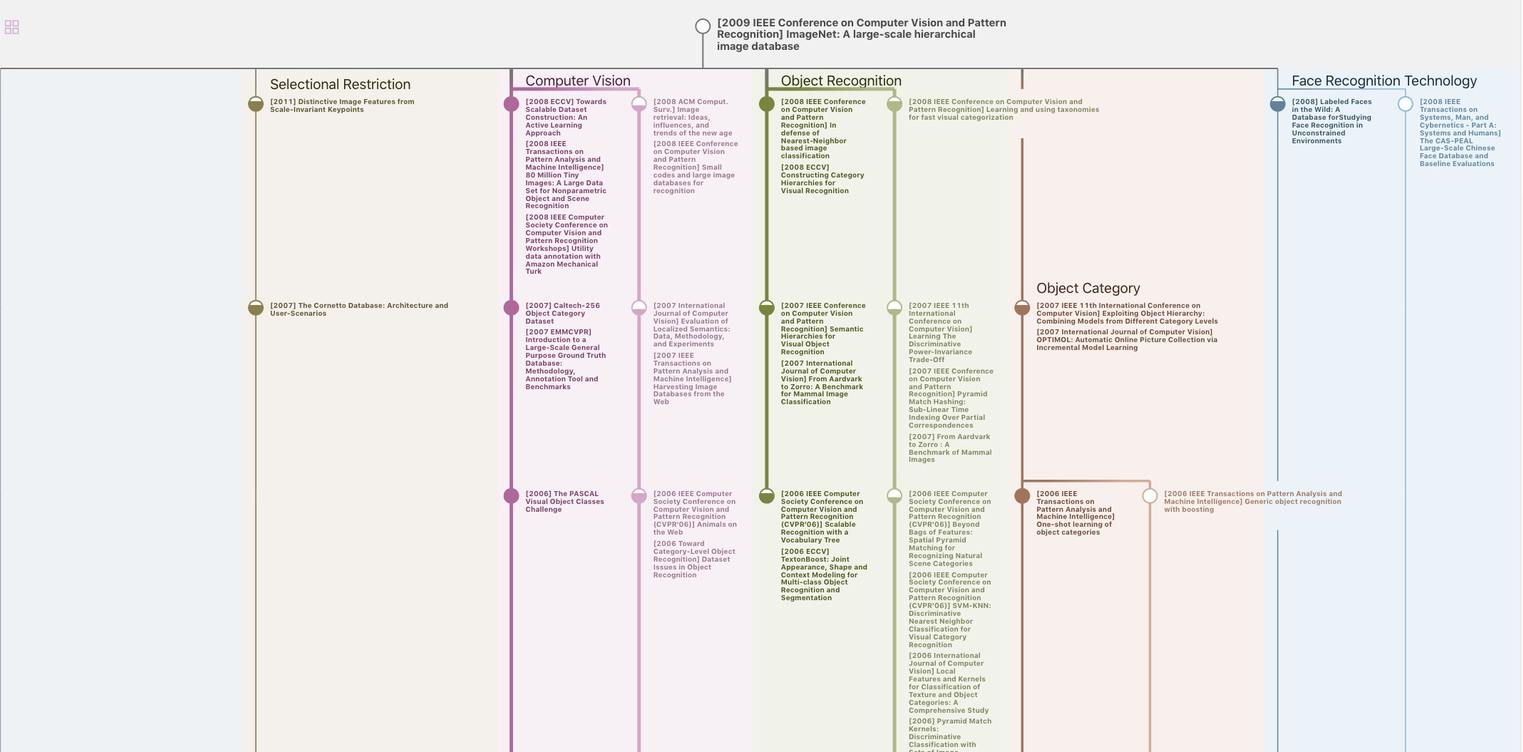
生成溯源树,研究论文发展脉络
Chat Paper
正在生成论文摘要