Editor's Evaluation: Neocortical Pyramidal Neurons with Axons Emerging from Dendrites Are Frequent in Non-Primates, but Rare in Monkey and Human
crossref(2022)
摘要
Article Figures and data Abstract Editor's evaluation Introduction Results Discussion Materials and methods Data availability References Decision letter Author response Article and author information Metrics Abstract The canonical view of neuronal function is that inputs are received by dendrites and somata, become integrated in the somatodendritic compartment and upon reaching a sufficient threshold, generate axonal output with axons emerging from the cell body. The latter is not necessarily the case. Instead, axons may originate from dendrites. The terms ‘axon carrying dendrite’ (AcD) and ‘AcD neurons’ have been coined to describe this feature. In rodent hippocampus, AcD cells are shown to be functionally ‘privileged’, since inputs here can circumvent somatic integration and lead to immediate action potential initiation in the axon. Here, we report on the diversity of axon origins in neocortical pyramidal cells of rodent, ungulate, carnivore, and primate. Detection methods were Thy-1-EGFP labeling in mouse, retrograde biocytin tracing in rat, cat, ferret, and macaque, SMI-32/βIV-spectrin immunofluorescence in pig, cat, and macaque, and Golgi staining in macaque and human. We found that in non-primate mammals, 10–21% of pyramidal cells of layers II–VI had an AcD. In marked contrast, in macaque and human, this proportion was lower and was particularly low for supragranular neurons. A comparison of six cortical areas (being sensory, association, and limbic in nature) in three macaques yielded percentages of AcD cells which varied by a factor of 2 between the areas and between the individuals. Unexpectedly, pyramidal cells in the white matter of postnatal cat and aged human cortex exhibit AcDs to much higher percentages. In addition, interneurons assessed in developing cat and adult human cortex had AcDs at type-specific proportions and for some types at much higher percentages than pyramidal cells. Our findings expand the current knowledge regarding the distribution and proportion of AcD cells in neocortex of non-primate taxa, which strikingly differ from primates where these cells are mainly found in deeper layers and white matter. Editor's evaluation Wahle and colleagues show that excitatory cortical neurons differ in the fundamental structural arrangement of dendrites, soma and axons across a range of mammalian species. Axons can originate directly from pyramidal cell dendrites in species as diverse as rodents, ferret, cats, pigs and primates. However, cross-species comparisons also indicate that non-primate brains have a higher proportion of axon-carrying-dendrites (AcD) than did brains of primates. This paper is of potential interest to a broad range of neuroscientists working on brain structure and function as well as computational models thereof. https://doi.org/10.7554/eLife.76101.sa0 Decision letter eLife's review process Introduction The prevailing concept of neocortical pyramidal cell function proposes that excitatory inputs arrive via the dendrites, are integrated in the somatodendritic compartment, and upon reaching sufficient threshold, the axonal domain generates an action potential. The axon usually originates from the ventral aspect of the soma, starting with a short axon hillock followed by the axon initial segment (AIS), the electrogenic domain generating the action potential (reviewed by Kole and Brette, 2018). Already Ramon y Cajal suggested that impulses may bypass the soma and flow directly to the axon (reviewed by Triarhou, 2014). Axon carrying dendrites (AcDs) are common in cortical inhibitory interneurons (Meyer, 1987; Wahle and Meyer, 1987; Meyer and Wahle, 1988; Höfflin et al., 2017). Furthermore, upright, inverted, and fusiform pyramidal neurons of supra- and infragranular layers display AcDs in Golgi impregnated or dye-injected cortex from rodents, lagomorphs, ungulates, and carnivores (Peters et al., 1968; Smit and Uylings, 1975; van der Loos, 1976; Peters and Kara, 1985; Ferrer et al., 1986a; Ferrer et al., 1986b; Hübener et al., 1990; Reblet et al., 1992; Matsubara et al., 1996; Prieto and Winer, 1999; Mendizabal-Zubiaga et al., 2007; Hamada et al., 2016; Ernst et al., 2018). In mouse hippocampal CA1 pyramidal cells, axons frequently emerge from basal dendrites (Thome et al., 2014). Multiphoton glutamate uncaging and patch clamp recordings revealed that input to the AcD is more efficient in eliciting an action potential than input onto regular dendrites (non-AcDs). AcDs are intrinsically more excitable, generating dendritic spikes with higher probability and greater strength. Synaptic input onto AcDs generates action potentials with lower thresholds compared to non-AcDs, presumably due to the short electrotonic distance between input and the AIS. The anatomical diversity of axon origins plus the diversity of length and position of the AIS substantially impact the electrical behavior of pyramidal neurons (reviewed by Kole and Brette, 2018). This begs the questions, of how frequent AcD pyramidal neurons are among the mammalian species, and whether AcD pyramidal neurons also exist in primates. Our data suggest remarkable differences between phylogenetic orders and position in gray and white matter. Results Pyramidal AcD cells in adult cortex We assigned AcDs in a very conservative manner. All cells in which the axonal origin could not be unequivocally seen to arise from a dendrite were considered ‘somatic’ axon cells. A certain fraction of neurons had an axon which shares a root with a dendrite. We consequently considered ‘shared root’ cells as somatic axon cells. Figure 1A documents the diversity of axon origins of pyramidal cells in a P60 infant macaque monkey cortex with an axon originating from a soma (inset B), an AcD (inset C), and the shared root configuration (inset D). Generally, AcDs were basal dendrites. AcD neurons of other species are shown in Figure 2A–E. Macaque neurons stained with SMI-32/βIV-spectrin are shown in three videos. Figure 2—video 1 shows an AcD pyramidal neuron from premotor cortex and flanking non-AcD neurons. Figure 2—video 2 shows a layer V pyramidal cell of the cingulate cortex with an axon wiggling out between thick dendrites at the right somatic pole. Even with the help of confocal imaging it was not easy to unequivocally identify the origin of the axon, and we score these neurons as a shared root cell. Figure 2—video 3 shows a spindle-shaped neuron resembling a Von Economo neuron of infragranular layers of the cingulate cortex. Note that the axon emerges >65 µm away from the soma from the descending dendrite. Figure 1 Download asset Open asset Confocal tile scan of dorsal neocortex (premotor area) of P60 infant macaque. (A) Pyramidal cells were stained with SMI-32/βIV-spectrin to label dendrites and the axon initial segment, respectively. Insets depict neurons with an axon emerging from the soma (B), or from an axon carrying dendrite (C), or a shared root (D). Axons indicated by arrows. Scale bars 100 µm for the tile scan and 25 µm for the insets. Figure 2 with 5 supplements see all Download asset Open asset Representative axon carrying dendrite (AcD) neurons. (A1, A2) From rat visual cortex (biocytin, immunofluorescence); (B1, B2) cat visual cortex (immunofluorescence); (C1, C2) ferret visual cortex (biocytin); (D1, D2) macaque premotor cortex (biocytin, immunofluorescence), the inset shows the axon origin at higher magnification; (E1, E2) human auditory cortex (Golgi method; D2 is a montage of two photos). Apical AcDs (asterisk in C2) were rare, less than 10 were detected among the neurons assessed in adult rat, ferret, and macaque, and none in our human material. In all cases, the axon immediately bent down toward the white matter. Axon origins are marked by large arrows, small arrows indicate the course of biocytin-labeled axons. Scale bars 25 µm. The online version of this article includes the following figure supplement for Figure 2: To further demonstrate the variability of the axon origin, Figure 2—figure supplement 1A-L shows representative biocytin-labeled neurons of rat, ferret, and macaque. The shared root configuration can be considered a transition between a clear-cut somatic axon and an axon originating from a dendrite. Neurons with the three features co-occur: for instance, of the group of rat pyramidal neurons depicted in Figure 2—figure supplement 1A and at higher magnifications in Figure 2—figure supplement 1B-D, some have somatic axons (cells labeled 1, 3), one has a shared root (cell 2) and two have AcDs (cells 4, 5). Figure 2—figure supplement 2A-O shows Golgi-impregnated pyramidal neurons of human cortex. The black reaction product made it more difficult to identify unequivocal AcD cells. Quantitative analysis In gray matter of non-primates, 10–21% of the pyramidal neurons assessed by perpendicular counts through all layers had an AcD (Figure 3A). The interindividual variability and staining methods are reported for mouse and rat in Table 1, and for cat, ferret, and pig in Table 2. Mouse Thy-1-EGFP, βIV-spectrin-positive pyramidal neurons vary from 10 to 22%, possibly due to the individual variability of the Thy-1 expression level. In adult macaque, gray matter of only about 3–6% of the pyramidal neurons had an AcD (Figure 3A). The interindividual variability and staining methods are reported in Table 3. In human gray matter, the proportion of AcD pyramidal neurons was 1.96% on average. The interindividual variability and staining methods are reported in Table 4. Figure 3 Download asset Open asset Proportion of axon carrying dendrite (AcD) neurons across species. (A) Shown are mean ± SEM. of the individual percentages listed in Tables 1–4, which also indicate the staining methods. Numbers above the bars are the total number of pyramidal neurons assessed per species/cell class for this graph. Numbers in the bars indicate the number of individuals. (B) Laminar analysis. Non-primate species showed roughly equal proportions of AcD neurons in supra- and infragranular layers. With some individual variability the range was 10–21%. In contrast, in macaque, the cluster was downshifted along the ordinate due to overall much lower proportions. Furthermore, infragranular pyramidal cells displayed much higher proportions of AcD cells compared with supragranular pyramidal cells. A Mann-Whitney rank sum test of ‘all non-primate’ versus ‘all macaque’ percentages of supragranular and infragranular AcD cells, yielded p<0.001 and p<0.001, respectively. Human was not included in the statistical test because only one method was used to detect AcD cells. The legend indicates the number of individuals and the staining methods; IFL, immunofluorescence. Note that we could not do a laminar analysis for all individuals shown in (A) because staining of supragranular layers in some animals delivered too low numbers which might have led to a sampling error. Figure 3—source data 1 Data and statistical analysis of experiments shown in Figure 3A, B. https://cdn.elifesciences.org/articles/76101/elife-76101-fig3-data1-v2.xlsx Download elife-76101-fig3-data1-v2.xlsx Table 1 Proportion of pyramidal neurons with AcD: rodents. Species; cortical area; staining method; age; sexProportion of AcD cells [%];n of cells assessedBy layers: supra %, infra %Mouse S1 cortex, layer V;Thy1-EGFP/βIV-spectrin immunofluorescenceAdult, female17.42%, 178 cellsn.a.Adult, female11.78%, 348 cellsn.a.Adult, female15.79%, 36 cellsn.a.Adult, female18.84%, 138 cellsn.a.Adult, male21.93%, 187 cellsn.a.Adult, male14.82%, 54 cellsn.a.Adult, male10.17%, 59 cellsn.a.Average [%], total n of cells15.82%, 1000 cellsRat visual cortex; biocytin tracingAdult, male, two hemispheres17.82%, 174 cells15.06%, 17.69%Adult, male, two hemispheres15.07%, 803 cells15.65%, 16.46%Average [%], total n of cells16.45%, 977 cells15.36%, 17.08% AcD, axon carrying dendrite; n.a., not applicable. Table 2 Proportion of pyramidal neurons with AcD: ungulate, carnivores. Species; cortical area; staining method; age; sexProportion of AcD cells [%];n of cells assessedBy layers: supra %, infra %Kitten visual cortex gyral white matter; intracellular Lucifer yellowP1, P2 (n = 2, sex n.d.)37.93%, 58 cellsn.a.P10, P11 (n = 2, sex n.d.)44.64%, 56 cellsn.a.P12, P14 (n = 2, sex n.d.)47.37%, 19 cellsn.a.Average [%], total n of cells43.31%, 133 cellsKitten and adult cat visual cortex layer VI; intracellular Lucifer yellowP1, P5 (n = 2, sex n.d.)20.18%, 114 cellsn.a.P11 (n = 2, sex n.d.)17.65%, 51 cellsn.a.P19, P30 (n = 2, sex n.d.)14.89%, 47 cellsn.a.P52, P60 (n = 2, sex n.d.)20.00%, 30 cellsn.a.Adult (n = 2, sex n.d.)17.95%, 39 cellsn.a.Average [%], total n of cells18.13%, 281 cellsAdult cat visual cortex;SMI-32/βIV-spectrin immunofluorescenceIndividual 1, 3 months, sex n.d.13.35%, 978 cells14.42%, 14.55%Individual 2, adult, sex n.d.14.92%, 496 cellsn.d.Adult cat visual cortex; biocytin tracingIndividual 3, adult, sex n.d.13.44%, 655 cells12.50%, 15.85%Individual 4, adult, sex n.d.17.14%, 70 cells18.52%, 12.50%Individual 5, adult, sex n.d.13.93%, 316 cells13.12%, 15.79%Individual 6, adult, sex n.d.19.13%, 230 cells19.34%,18.37%Individual 7, adult, sex n.d.12.64%, 174 cells11.49 %, 19.23%Average [%] individuals 1–7, total n of cells14.94%, 2919 cells14.90%, 16.05%Ferret visual cortex; biocytin tracingIndividual 1, adult, female16.56%, 302 cells15.95%, 18.57%Individual 2, adult, female14.66%, 191 cells15.39%, 13.11%Individual 3, adult, female11.30%, 230 cells10.24%, 14.06%Individual 4, adult, male13.07%, 329 cells13.45%, 12.26%Average [%], total n of cells13.90%, 1052 cells13.76%, 14.50%Pig dorsoparietal cortex;SMI-32/βIV-spectrin immunofluorescence3 months, European wild boar, female20.11%, 189 cells22.04%, 19.05%5 months, domestic, sex n.d.20.99%, 181 cellsn.d.Average [%], total n of cells20.55%, 370 cells22.04%, 19.05% AcD, axon carrying dendrite; n.a., not applicable; n.d., not determined due to too weak staining. Table 3 Proportion of pyramidal neurons with AcD: primates - macaque. Species; cortical area; staining method; age; sexProportion of AcD cells [%];n of cells assessedBy layers: supra %, infra %Macaca mulatta premotor cortex; biocytin tracingIndividual 1, 11 years, male4.93%, 954 cells2.79%, 11.38%Individual 2, 7 years, male3.10%, 816 cells2.93%, 6.32%Individual 3, 5 years, male4.26%, 423 cells3.23%, 6.25%Macaca mulatta parietal and visual cortex; SMI-32/βIV-spectrin immunofluorescenceIndividual 4, 5 years, male5.99%, 717 cells2.59%, 13.95%Individual 5, 10 years, male6.75%, 681 cells2.51%, 12.72%Macaca fascicularis parietal cortex;Golgi-Kopsch methodIndividual 6, adult, female3.58%, 307 cells4.82%, 6.90%Individual 7, adult, male3.07%, 228 cells1.57%, 5.67%Average [%] individuals 1–7, total n of cells4.53%, 4126 cells2.92%, 9.03%Macaca mulatta visual cortex; biocytin tracingIndividual 8, P60, female4.51%, 377 cells1.28%, 5.88%Macaca mulatta cingulate cortex;SMI-32/βIV-spectrin immunofluorescenceIndividual 8, P60, female5.80%, 500 cells1.28%, 7.85%Macaca mulatta premotor/M2 cortex;SMI-32/βIV-spectrin immunofluorescenceIndividual 8, P60, female5.36%, 1249 cells1.34%, 9.05%Average [%] individual 8, total n of cells5.22%, 2126 cells1.30%, 7.59% AcD, axon carrying dendrite. Table 4 Proportion of pyramidal neurons with AcD: primates - human. Species; cortical area; staining method; age; sexProportion of AcD cells [%];n of cells assessedBy layers: supra %, infra %Human temporal lobe;Golgi-Cox methodIndividual 1, 53 years, male2.56%, 646 cells1.45%, 4.40%Individual 2, 56 years, male2.79%, 825 cells0.96%, 4.66%Human auditory cortex;Golgi-Kopsch methodIndividual 3, 63 years, male0.79%, 253 cells0.69%, 0.91%Individual 4, 71 years, female1.92%, 677 cells0.80%, 2.58%Individual 5, 75 years, male0.47%, 215 cells0.00%, 0.80%Individual 6, 88 years, female0.71%, 140 cells0.00%, 1.28%Individual 7, 56 years, female1.72%, 407 cells1.27%, 2.34%Human prefrontal agranular cortex;Golgi-Kopsch methodIndividual 8, 46 years, female2.02%, 247 cellsn.d.Individual 9, 77 years, male2.59%, 424 cellsn.d.Individual 10, 87 years, female1.53%, 653 cellsn.d.Human visual cortex area 18Golgi-Kopsch methodIndividual 10, 87 years, female2.48%, 242 cells1.77%, 3.10%Average [%] individuals 1–10, total n of cells1.96%, 4729 cells0.99%, 2.87%Human auditory cortex gyral white matter;Golgi-Kopsch methodIndividual 3, 63 years, male8.69%, 115 cells,n.a.Individual 4, 71 years, female8.88%, 135 cells,n.a.Individual 5, 75 years, male8.70%, 69 cells,n.a.Individual 6, 88 years, female9.18%, 98 cells,n.a.Average [%] individuals 3–6, total n of cells8.86%, 417 cellsn.a. AcD, axon carrying dendrite. A significant difference emerged after a layer-specific analysis. Proportions were largely obtained in a second round of quantification with surface-parallel tracks, and in some cases sections were assessed that had not been analyzed in the first round of counting in order to obtain higher cell numbers. Therefore, in Tables 1–4 the laminar percentages do not simply add up to proportions obtained for whole gray matter. Furthermore, we plotted the individual values because bar graphs do not represent interindividual variability. Non-primates had about equal proportions of AcD cells in supra- and infragranular layers (Figure 3B, Tables 1 and 2). Thy-1 was only expressed in layer V and therefore, did not allow to determine laminar percentages for mouse. The macaque had only about 1–5% supragranular and about 5–14% infragranular AcD cells (Figure 3B, Table 3). Note the variable proportions of AcD neurons in infragranular layers and no obvious correlation between proportion and age of the individual macaques. The differences between non-primates and macaques were significant (see legend to Figure 3B). Values obtained in human Golgi material overlap with the lower range of the macaque values. Also in humans, supragranular layers had low proportions of AcD cells of 0.99% on average. Laminar percentages for infragranular neurons were on average 2.87%, and variable between individuals, but obviously not correlated with age (Table 4). Note that levels might have been a bit underestimated in Golgi material. The point will be addressed below. For a more detailed analysis, we compared six cortical areas (primary sensory to limbic) in macaque using the same method, SMI-32/βIV-spectrin immunofluorescence. Antibody SMI-32 is directed against nonphosphorylated neurofilaments. It labels somata and dendrites of large type 1 pyramidal cells mainly of layers III and V, and much weaker the smaller pyramidal neurons, but not spiny stellates of layer IV and small pyramidal neurons of layer II (García-Cabezas and Barbas, 2014). βIV-spectrin is one of the most reproducible markers for the AIS. The following regions were assessed: visual cortex V1/operculum, auditory cortex A1 along the lower bank of the lateral fissure, somatosensory cortex S2 along the upper bank of the lateral fissure, cingulate cortex medial and lateral flank including areas 23 and 31, respectively, the upper and lower bank of the intraparietal sulcus, and dorsal cortex (premotor and parietal at more anterior levels). The intraparietal sulcus has on the upper bank area MIP (medial intraparietal area) involved in grasping, and on the lower bank the areas LIP (lateral intraparietal area) and VIP (ventral intraparietal area) involved in control of eye movements. Intraparietal neurons were retrogradely labeled from the premotor cortex injections. In three individuals, the percentages of AcD neurons varied between the six areas by about a factor of 2 (Figure 4A). We could not recognize a systematic difference in that one of the areas presented with substantially higher or lower percentages. Figure 4 Download asset Open asset Within-species areal comparisons. (A) Upper left is a photomicrograph of one of the coronal sections stained for immunofluorescence. The regions of interest are color coded. Upper right is the macaque brain (after Paxinos et al., 2009). The dashed boxes and Bregma distances indicate where our assessments were made. The rostral box overlaps the premotor cortex harboring the biocytin injections. Note that the analysis was spanning several millimeters of cortex (see Figure 4—source data 1). The middle box corresponds to the level of the section shown to the left. It is slightly tilted with respect to the stereotaxis coordinates (Paxinos et al., 2009). The posterior box corresponds to a fairly caudal level of the visual cortex. The table summarizes the percentages of AcD neurons obtained in the six areas and three individuals and gives the mean of each area with standard deviation. Abbreviations: arc, arcuate sulcus; cgs, cingulate sulcus; cs, central sulcus; ecal, external calcarine sulcus; ios, inferior occipital sulcus; ips, intraparietal sulcus; lf, lateral fissure; lu, lunate sulcus; prs, principal sulcus; sts, superior temporal sulcus. (B) Upper left is a photomicrograph of one of the coronal sections of cat occipital cortex analyzed for biocytin-stained AcD neurons. The injection site in this case was near the area 17/18 border, some other cats had an additional injection into the suprasylvian gyrus (see Figure 4—source data 1). Area 17 is along the medial flank, areas 18, 19, and 21 are in the lateral sulcus and on the suprasylvian gyrus. Upper right is the cat brain (after Reinoso-Suarez, 1961) with the visual fields indicated. The table summarizes the percentages of AcD neurons obtained in area 17 and the extrastriate areas. The graph pairs the data points of the five cats. To the right, we compared cat (n = 7) to ferret (n = 4) visual cortex (striate and extrastriate). Every point is one individual, the red bar represents the median for each column. The p-values were determined with a Mann-Whitney rank sum test. Figure 4—source data 1 Data and statistical analysis of experiments shown in Figure 4A and B. https://cdn.elifesciences.org/articles/76101/elife-76101-fig4-data1-v2.xlsx Download elife-76101-fig4-data1-v2.xlsx Furthermore, we compared primary visual area 17 to extrastriate visual areas in adult cats which received biocytin injections. Also here, individual percentages of AcD neurons varied from 11 to 20%; the interindividual variability was larger than the interareal variability. There was no recognizable difference between the areas analyzed (Figure 4B). Moreover, the values obtained in cat visual cortex matched those in ferret visual cortex (striate and extrastriate) (Figure 4B; Table 2). As defined in the beginning, all neurons in which the axon origin was not unequivocally seen to emerge from a dendrite were scored as shared root cells, and for Figure 3 and Figure 4 were included in the group of cells with somatic axons. Yet, cells with the shared root configuration according to our criteria have been accepted in recent studies already as AcD neurons (Thome et al., 2014). The question was, how often does the shared root configuration according to our definition occur? We plotted the percentages of AcD neurons versus shared root neurons for rat, ferret, macaque, and human (Figure 5A). For macaque, we included the biocytin-stained material from premotor cortex. In macaque Individual 2 we could assess the contralateral cortex to determine the percentage of AcD and shared root cells of callosal projection neurons. Furthermore, in Individual 2, long-range projection neurons residing in the intraparietal sulcus were assessed, which in functional terms belong to the eye-hand coordination and grasping network. Furthermore, we determined the shared root configuration in all areas shown in Figure 4A visualized via immunofluorescence. Also included were the values obtained in Golgi-stained macaque and human cortex (see Figure 5—source data 1). In Figure 5A, the species cluster along the ordinate as already evident in Figure 3B. However, in Figure 5A, the species scatter widely along the abscissa. This suggested an absence of a systematic correlation between AcD and the shared root configuration. Figure 5 Download asset Open asset Proportion of axon carrying dendrite (AcD) cells versus shared root cells. (A) Data from rat (biocytin), ferret (biocytin), macaque (biocytin, immunofluorescence, Golgi), and human (Golgi). The species cluster along the ordinate as already seen in Figure 3B. The Mann-Whitney rank sum test of ‘all non-primate’ versus ‘all macaque’ proportions of AcD cells yielded p <0.001. However, the shared root values scatter considerably along the abscissa. Mann-Whitney rank sum test of ‘all non-primate’ versus ‘all macaque’ proportions of shared root cells yielded p=0.008. (B) The percentages of AcD were graphically compared to the sum of AcD and shared root. For macaque, data were separated by staining methods. Note that the Golgi method in macaque and in human yielded a higher proportion of shared root compared to biocytin and immunofluorescence. Numbers in the bars represent the sample size (individuals and/or cortical areas). (C) Comparison of biocytin and immunofluorescence staining in macaque. (D) Comparison of biocytin and immunofluorescence staining within just one individual macaque. Note in C, D that AcD cells are detected equally well with both methods whereas the biocytin staining yielded higher numbers of shared root cells (SR). In C, D, colors indicate the comparisons, and the p-values were determined with a Mann-Whitney rank sum test. IFL, immunofluorescence. Figure 5—source data 1 Data and statistical analysis of experiments shown in Figure 5A and B. https://cdn.elifesciences.org/articles/76101/elife-76101-fig5-data1-v2.xlsx Download elife-76101-fig5-data1-v2.xlsx Next, for rat, ferret, macaque, and human, we compared the percentages of AcD to the sum of AcD plus shared root (Figure 5B). If the shared root cells were considered as AcD cells, the proportions of AcD cells increase to some extent in all species analyzed. The interindividual variability of the shared root cells was at a factor of >10 (range of 0.46–5.5% in macaque), and statistics argued against any biologically significant difference between species. Unexpectedly, a subtle difference was observed independently by two observers who analyzed the Golgi material (PW at Ruhr University Bochum, GM at University La Laguna). A total of 13 cases (2 macaque, 11 human individuals) had percentages of shared root cells higher than percentages of AcD cells, whereas in 22 of 25 individuals and/or cortical areas stained for biocytin and immunofluorescence the percentages of shared root cells were lower than the percentages of AcD cells (Figure 5B). Thus, the proportion of AcD neurons was slightly underrepresented in the Golgi material. Yet, also the biocytin material had larger proportions of shared root cells (Figure 5B). We therefore compared immunofluorescence and biocytin of the macaque material (Figure 5C). Indeed, the biocytin material delivered significantly more shared root cells. The unequivocal AcD cells were equally well recognized by both methods. With the large data set of macaque Individual 2 we compared the two methods within one individual (Figure 5D). Again, similar to the Golgi material, the proportion of shared root cells was higher in the biocytin material than it was in the immunofluorescence material whereas unequivocal AcD cells were equally well detected with the two methods. AcDs in rodent hippocampus are described as being functionally privileged. This may be mirrored by their spine density. Analysis of rat and ferret biocytin-stained pyramidal cells, however, revealed that neither the dendrites sharing a root with an axon nor the AcDs had spine densities differing systematically from the spine density of non-AcDs from the very same neuron (Figure 6). Figure 6 Download asset Open asset Spine density did not systematically differ between regular dendrites (non-axon carrying dendrites [non-AcDs]), dendrites sharing a root with a neighboring axon, and AcDs. Data from adult rat and ferret biocytin material, values from each cell are connected by a line. For normalization, the average of the non-AcD has been set to 1, and all values were expressed relative to this. Mann-Whitney rank sum test p-values and the sample size are reported above each plot. Figure 6—source data 1 Data and statistical analysis of experiments shown in Figure 6. https://cdn.elifesciences.org/articles/76101/elife-76101-fig6-data1-v2.xlsx Download elife-76101-fig6-data1-v2.xlsx Developmental aspects Kitten layer VI pyramidal cells (Figure 3A, Table 2) showed adult percentages of AcDs early postnatally. Many pyramidal cells were L-shaped or inverted-fusiform, with the axon emerging from one of the dominant dendrites (Lübke and Albus, 1989). In line with this, infant macaque cortex exhibited percentages of AcD cells comparable to adult cortex neurons labeled with biocytin, and again, AcD cells were more frequent in infragranular layers (Figure 3A and B, Table 3). Unexpectedly, of the pyramidal cells in kitten white matter (Wahle et al., 1994), 43.31% had axons emerging from the major dendrite (Table 2). Even more striking, 8.86% of the interstitial pyramidal neurons of the adult human white matter (Meyer et al., 1992) displayed AcDs (Table 4), and on average an additional 13.23% of the interstitial pyramidal cells had axons emerging from a shared root. Interneurons We also assessed the proportion of AcD of interneurons. In human Golgi material, interneurons were easily recognized by non-spiny slightly varicose dendrites, lack of polarity, and – if present – locally branching axons. The more grazile morphology with ra
更多查看译文
关键词
Neural Development,Cortical Development,Axon guidance
AI 理解论文
溯源树
样例
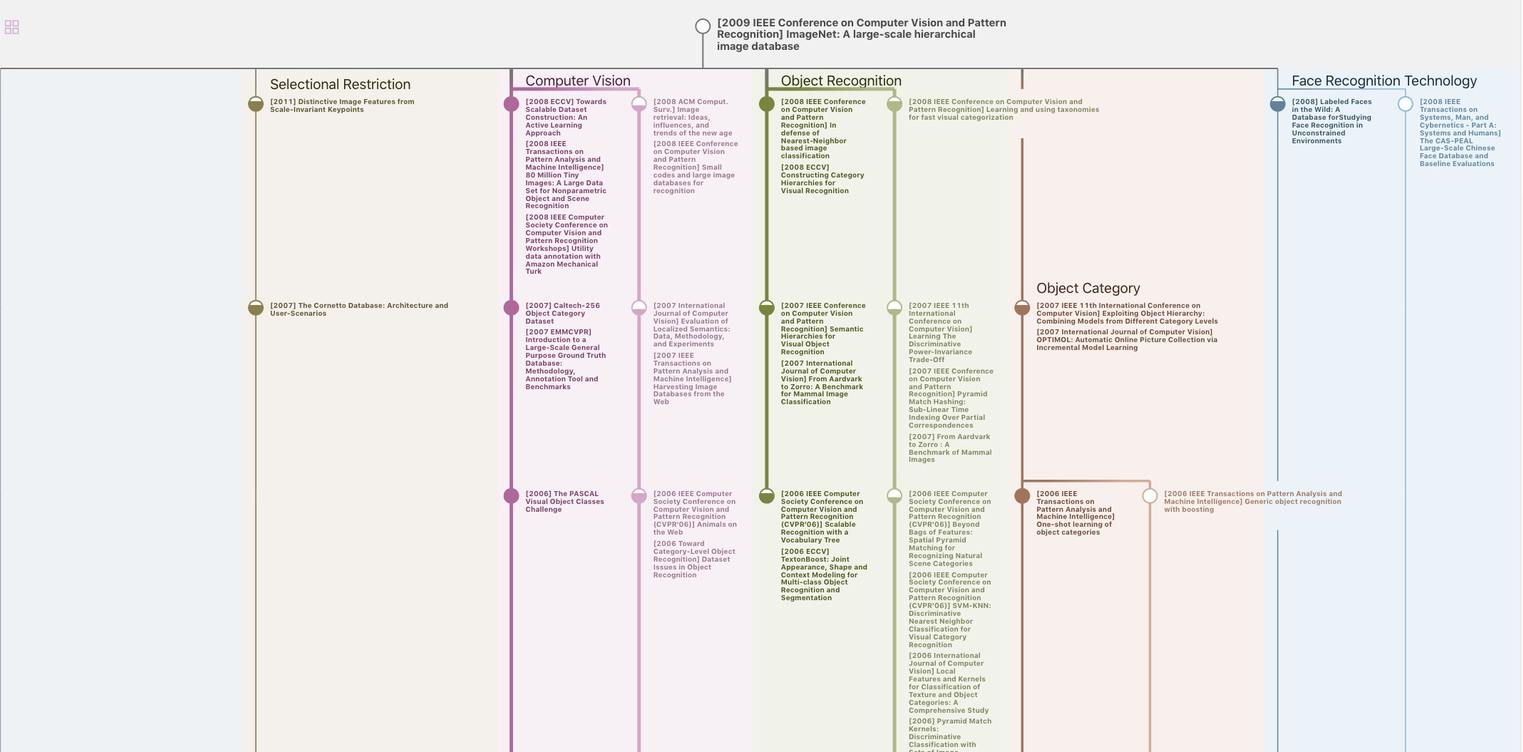
生成溯源树,研究论文发展脉络
Chat Paper
正在生成论文摘要