The primary target facilityfor a neutrino factorybased on muon beamsa
semanticscholar(2007)
Abstract
Neutrino beams from the decay of muons in a storage ring o er the prospect of very high ux, well-understood spectra, and equal numbers of electron and muon neutrinos, as desirable for detailed exploration of neutrino oscillations via long baseline detectors [1]. Such beams require large numbers of muons, and hence a high performance target station at which a 1-4 MW proton beam of 16-24 GeV impinges on a compact target, all inside a high eld solenoid channel to capture as much of the phase volume of soft pions as possible. A rst concept was based on a carbon target, as reported in 2000 the Neutrino Factory Feasibility Study-I [2]. A higher performance option based on a free mercury jet has been studied in 2001 as part of the Neutrino Factory Feasibility Study-II [3, 4]. An overview of a mercury jet target facility is presented here, including requirements, design concept and summaries of simulated performance. Further details are presented in related papers at this conference. 1 THE TARGET FACILITY A muon collider [18] or a neutrino factory based on a muon storage ring [1, 2, 3, 4] require intense beams of muons, which are obtained from the decay of pions produced in proton-nucleus collisions. To maximize the yield, pions of momentum near 300 MeV/c should be captured, as illustrated in Fig. 1. For proton energies above 10 GeV, the pion yield per unit of proton beam energy is larger for a high-Z target [5]. For proton beam energies in the MW range, beam heating would melt/boil a stationary high-Z target, so a moving target must be used. A mercury jet target is the main option considered here, although several alternatives remain under active study [6, 7]. For greater detail, consult Chap. 3 of [3]. See also [8]. The low-energy pions are produced with relatively large angles to the proton beam, and e cient capture into a decay and phase rotation channel [9] is obtained by surrounding the target with a 20-T solenoid magnet, whose eld tapers down to 1.25 T over several meters, as sketched in Fig. 2. Pion yield is 2 Figure 1: Comparison of pion yield measured in BNL E910 with a MARS calculation. maximized with a mercury target in the form a 1-cm-diameter cylinder, tilted by about 100 mrad with respect to the magnetic axis. To permit the proton beam to interact with the target over 2 interaction lengths, the proton beam is tilted by 33 mrad with respect to the mercury jet axis. See also Fig. 3. A mercury pool inside the capture solenoid intercepts the mercury jet and the unscattered proton beam, as shown in Fig. 4. The mercury pool, surrounding tungsten carbide/water shielding, and the resistive insert of the 20-T capture magnet [10] are isolated from upstream and downstream beamline elements by a pair of double-walled Be windows. This entire unit can be replaced by remote manipulation should failure occur. The absorbed radiation dose on components 3 Figure 2: Sketch of the target and capture system based on a mercury jet inside a 20-T solenoid magnet. near the target is quite large [5], as illustrated in Fig. 5, such that in a 4 Mw proton beam, their lifetime against radiation damage may only be 5 years. The capture solenoid is encased in thick concrete shielding as part of the target facility that includes an overhead crane, hot cells with remote manipulation capability, and a mercury pumping and puri cation loop [11], as sketched in Fig. 6. The use of a mercury jet target raises several novel issues. The rapid energy deposition in the mercury target by the proton beam leads to intense pressure waves that can disperse the mercury [12, 13, 14]. Further, as the mercury enters the strong magnetic eld eddy currents are induced in the mercury, and the Lorentz force on these currents could lead to distortion of the jet [15, 16]. On the other hand, the magnetic pressure on the mercury once inside the solenoid will damp mechanical perturbation of the jet. An R&D program is underway to assess these critical issues [17]. 4 Figure 3: The inner region of the 20-T capture magnet along with the tilted mercury jet target and proton beam. 5 Figure 4: A mercury pool inside the capture solenoid intercepts the mercury jet and the unscattered proton beam. 6 FS−2 24 GeV Target Station: MARS14 02/19/01 R, Z, Z X 0 35 70 105 140 cm 0 200 400 600 600 cm 105 104 103 102 101 100 10−1 10−2 10−3 3.6e+06 0.0e+00 Azimuthally averaged absorbed dose (MGy/yr) Figure 5: Absorber radiation dose in the pion capture system per 2 107 s of a 1-MW, 24-GeV proton beam on a mercury target. 7 Figure 6: Sketch of the target facility. 8 2 References [1] C. Albright et al., Physics at a Neutrino Factory, hep-ex/0008064. [2] N. Holtkamp, D. Finley, eds., A Feasibility Study of a Neutrino Source Based on A Muon Storage Ring, http://www.fnal.gov/projects/muon collider/nu-factory/ [3] S. Ozaki et al., eds., Feasibility Study-II of a Muon-Based Neutrino Source, http://www.cap.bnl.gov/mumu/studyii/FS2-report.html [4] R. Palmer et al., A Second Feasibility Study of a Muon Storage Ring Neutrino Factory, PAC2001, FOAC007. [5] N. Mokhov, Particle Production and Radiation Environment at a Neutrino Factory Target Station, PAC2001, FOAC010. [6] B. King et al., An Inconel Rotating Band Pion Production Target for Neutrino Factories and Muon Colliders, PAC2001, TPAH136. [7] V. Balbekov and N. Mokhov, Low-Budget Muon Source, PAC2001, TPAH144. [8] T. Gabriel et al., Targets for High-Intensity Particle Production, PAC2001, FOAC008. [9] H. Kirk et al., Phase Rotation at the Front End of a Neutrino Factory, PAC2001, TPPH161. [10] R. Weggel et al., Design Study for 20 T, 15 cm Bore Hybrid Magnet with Radiation-Resistant Insert for Pion Capture, PAC2001, RPPH074. [11] P. Spampinato et al., Target Enclosure/System Design for a MercuryTarget Neutrino Producing Facility, PAC2001, TPAH154. 9 [12] N. Simos et al., Thermodynamic Interaction of the Primary Proton Beam with a Mercury Jet Target at a Neutrino Factory Source, PAC2001, RPAH076. [13] A. Hassanein and I. Konkashbaev, Thermoelastic Response of Suddenly Heated Liquid and Solid Targets for High Power Colliders, PAC2001, RPAH071. [14] R. Samulyak and W. Oh, Global Numerical Modeling of the Muon Collider Target, PAC2001, TPAH139. [15] S. Kahn et al., Calculations for a Mercury Jet Target in a Solenoid Magnet Capture System, PAC2001, ROPB010. [16] A. Hassanein and I. Konkashbaev, Dynamics of Liquid Metal Jets Penetrating a Strong Magnetic Field, PAC2001, ROPB009. [17] K. Brown et al., The R&D Program for Targetry at a Neutrino Factory, PAC2001, TPAH156. [18] C.M. Ankenbrandt et al. (The Muon Collider Collaboration), Status of muon collider research and development and future plans, Phys. Rev. ST Accel. Beams 2, 081001 (1999), http://prstab.aps.org/abstract/PRSTAB/V2/i8/E081001/ 10
MoreTranslated text
AI Read Science
Must-Reading Tree
Example
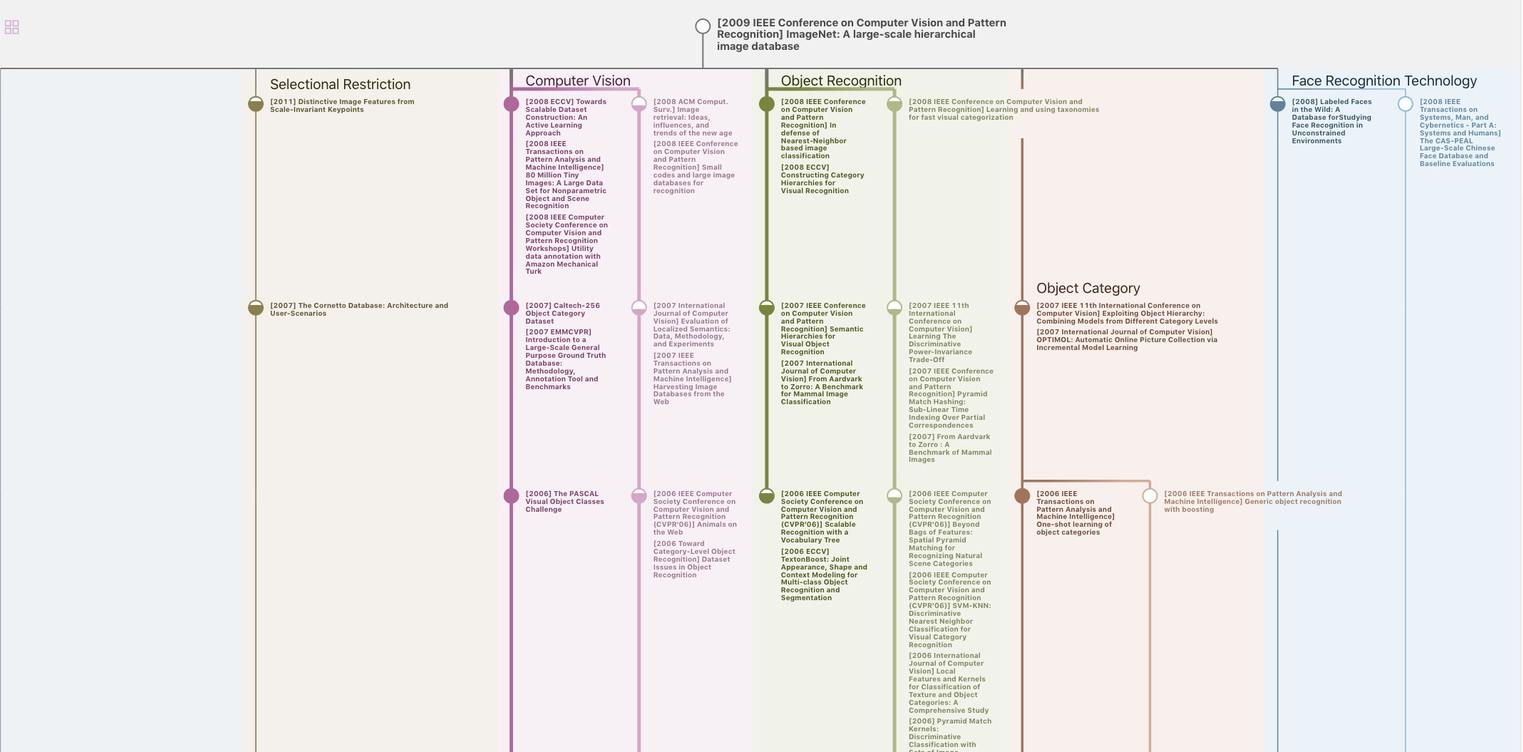
Generate MRT to find the research sequence of this paper
Chat Paper
Summary is being generated by the instructions you defined