HNF1A recruits KDM6A to activate differentiated acinar cell programs that suppress pancreatic cancer
The EMBO Journal(2020)
摘要
Article10 March 2020Open Access Transparent process HNF1A recruits KDM6A to activate differentiated acinar cell programs that suppress pancreatic cancer Mark Kalisz Mark Kalisz orcid.org/0000-0002-8770-2798 Section of Epigenomics and Disease, Department of Medicine, Imperial College London, London, UK Epithelial Carcinogenesis Group, Spanish National Cancer Research Centre-CNIO, Madrid, Spain CIBERONC, Madrid, Spain Search for more papers by this author Edgar Bernardo Edgar Bernardo Bioinformatics and Genomics Program, Centre for Genomic Regulation (CRG), The Barcelona Institute of Science and Technology (BIST), Barcelona, Spain Centro de Investigación Biomédica en Red de Diabetes y Enfermedades Metabólicas Asociadas (CIBERDEM), Barcelona, Spain Search for more papers by this author Anthony Beucher Anthony Beucher Section of Epigenomics and Disease, Department of Medicine, Imperial College London, London, UK Search for more papers by this author Miguel Angel Maestro Miguel Angel Maestro Bioinformatics and Genomics Program, Centre for Genomic Regulation (CRG), The Barcelona Institute of Science and Technology (BIST), Barcelona, Spain Centro de Investigación Biomédica en Red de Diabetes y Enfermedades Metabólicas Asociadas (CIBERDEM), Barcelona, Spain Search for more papers by this author Natalia del Pozo Natalia del Pozo Epithelial Carcinogenesis Group, Spanish National Cancer Research Centre-CNIO, Madrid, Spain CIBERONC, Madrid, Spain Search for more papers by this author Irene Millán Irene Millán Epithelial Carcinogenesis Group, Spanish National Cancer Research Centre-CNIO, Madrid, Spain CIBERONC, Madrid, Spain Search for more papers by this author Lena Haeberle Lena Haeberle orcid.org/0000-0002-8793-568X Institute of Pathology, Heinrich-Heine University and University Hospital of Düsseldorf, Düsseldorf, Germany Search for more papers by this author Martin Schlensog Martin Schlensog Institute of Pathology, Heinrich-Heine University and University Hospital of Düsseldorf, Düsseldorf, Germany Search for more papers by this author Sami Alexander Safi Sami Alexander Safi Department of Surgery, Heinrich-Heine University and University Hospital of Düsseldorf, Düsseldorf, Germany Search for more papers by this author Wolfram Trudo Knoefel Wolfram Trudo Knoefel Department of Surgery, Heinrich-Heine University and University Hospital of Düsseldorf, Düsseldorf, Germany Search for more papers by this author Vanessa Grau Vanessa Grau Bioinformatics and Genomics Program, Centre for Genomic Regulation (CRG), The Barcelona Institute of Science and Technology (BIST), Barcelona, Spain Centro de Investigación Biomédica en Red de Diabetes y Enfermedades Metabólicas Asociadas (CIBERDEM), Barcelona, Spain Search for more papers by this author Matías de Vas Matías de Vas Section of Epigenomics and Disease, Department of Medicine, Imperial College London, London, UK Search for more papers by this author Karl B Shpargel Karl B Shpargel Department of Genetics and Lineberger Comprehensive Cancer Center, University of North Carolina at Chapel Hill, Chapel Hill, NC, USA Search for more papers by this author Eva Vaquero Eva Vaquero CiberEHD, Institut de Malalties Digestives i Metabòliques, Hospital Clínic, IDIBAPS, Barcelona, Spain Search for more papers by this author Terry Magnuson Terry Magnuson Department of Genetics and Lineberger Comprehensive Cancer Center, University of North Carolina at Chapel Hill, Chapel Hill, NC, USA Search for more papers by this author Sagrario Ortega Sagrario Ortega Transgenics Unit, Spanish National Cancer Research Centre-CNIO, Madrid, Spain Search for more papers by this author Irene Esposito Irene Esposito Department of Surgery, Heinrich-Heine University and University Hospital of Düsseldorf, Düsseldorf, Germany Search for more papers by this author Francisco X Real Francisco X Real Epithelial Carcinogenesis Group, Spanish National Cancer Research Centre-CNIO, Madrid, Spain CIBERONC, Madrid, Spain Departament de Ciències Experimentals i de la Salut, Universitat Pompeu Fabra, Barcelona, Spain Search for more papers by this author Jorge Ferrer Corresponding Author Jorge Ferrer [email protected] orcid.org/0000-0002-5959-5729 Section of Epigenomics and Disease, Department of Medicine, Imperial College London, London, UK Bioinformatics and Genomics Program, Centre for Genomic Regulation (CRG), The Barcelona Institute of Science and Technology (BIST), Barcelona, Spain Centro de Investigación Biomédica en Red de Diabetes y Enfermedades Metabólicas Asociadas (CIBERDEM), Barcelona, Spain Search for more papers by this author Mark Kalisz Mark Kalisz orcid.org/0000-0002-8770-2798 Section of Epigenomics and Disease, Department of Medicine, Imperial College London, London, UK Epithelial Carcinogenesis Group, Spanish National Cancer Research Centre-CNIO, Madrid, Spain CIBERONC, Madrid, Spain Search for more papers by this author Edgar Bernardo Edgar Bernardo Bioinformatics and Genomics Program, Centre for Genomic Regulation (CRG), The Barcelona Institute of Science and Technology (BIST), Barcelona, Spain Centro de Investigación Biomédica en Red de Diabetes y Enfermedades Metabólicas Asociadas (CIBERDEM), Barcelona, Spain Search for more papers by this author Anthony Beucher Anthony Beucher Section of Epigenomics and Disease, Department of Medicine, Imperial College London, London, UK Search for more papers by this author Miguel Angel Maestro Miguel Angel Maestro Bioinformatics and Genomics Program, Centre for Genomic Regulation (CRG), The Barcelona Institute of Science and Technology (BIST), Barcelona, Spain Centro de Investigación Biomédica en Red de Diabetes y Enfermedades Metabólicas Asociadas (CIBERDEM), Barcelona, Spain Search for more papers by this author Natalia del Pozo Natalia del Pozo Epithelial Carcinogenesis Group, Spanish National Cancer Research Centre-CNIO, Madrid, Spain CIBERONC, Madrid, Spain Search for more papers by this author Irene Millán Irene Millán Epithelial Carcinogenesis Group, Spanish National Cancer Research Centre-CNIO, Madrid, Spain CIBERONC, Madrid, Spain Search for more papers by this author Lena Haeberle Lena Haeberle orcid.org/0000-0002-8793-568X Institute of Pathology, Heinrich-Heine University and University Hospital of Düsseldorf, Düsseldorf, Germany Search for more papers by this author Martin Schlensog Martin Schlensog Institute of Pathology, Heinrich-Heine University and University Hospital of Düsseldorf, Düsseldorf, Germany Search for more papers by this author Sami Alexander Safi Sami Alexander Safi Department of Surgery, Heinrich-Heine University and University Hospital of Düsseldorf, Düsseldorf, Germany Search for more papers by this author Wolfram Trudo Knoefel Wolfram Trudo Knoefel Department of Surgery, Heinrich-Heine University and University Hospital of Düsseldorf, Düsseldorf, Germany Search for more papers by this author Vanessa Grau Vanessa Grau Bioinformatics and Genomics Program, Centre for Genomic Regulation (CRG), The Barcelona Institute of Science and Technology (BIST), Barcelona, Spain Centro de Investigación Biomédica en Red de Diabetes y Enfermedades Metabólicas Asociadas (CIBERDEM), Barcelona, Spain Search for more papers by this author Matías de Vas Matías de Vas Section of Epigenomics and Disease, Department of Medicine, Imperial College London, London, UK Search for more papers by this author Karl B Shpargel Karl B Shpargel Department of Genetics and Lineberger Comprehensive Cancer Center, University of North Carolina at Chapel Hill, Chapel Hill, NC, USA Search for more papers by this author Eva Vaquero Eva Vaquero CiberEHD, Institut de Malalties Digestives i Metabòliques, Hospital Clínic, IDIBAPS, Barcelona, Spain Search for more papers by this author Terry Magnuson Terry Magnuson Department of Genetics and Lineberger Comprehensive Cancer Center, University of North Carolina at Chapel Hill, Chapel Hill, NC, USA Search for more papers by this author Sagrario Ortega Sagrario Ortega Transgenics Unit, Spanish National Cancer Research Centre-CNIO, Madrid, Spain Search for more papers by this author Irene Esposito Irene Esposito Department of Surgery, Heinrich-Heine University and University Hospital of Düsseldorf, Düsseldorf, Germany Search for more papers by this author Francisco X Real Francisco X Real Epithelial Carcinogenesis Group, Spanish National Cancer Research Centre-CNIO, Madrid, Spain CIBERONC, Madrid, Spain Departament de Ciències Experimentals i de la Salut, Universitat Pompeu Fabra, Barcelona, Spain Search for more papers by this author Jorge Ferrer Corresponding Author Jorge Ferrer [email protected] orcid.org/0000-0002-5959-5729 Section of Epigenomics and Disease, Department of Medicine, Imperial College London, London, UK Bioinformatics and Genomics Program, Centre for Genomic Regulation (CRG), The Barcelona Institute of Science and Technology (BIST), Barcelona, Spain Centro de Investigación Biomédica en Red de Diabetes y Enfermedades Metabólicas Asociadas (CIBERDEM), Barcelona, Spain Search for more papers by this author Author Information Mark Kalisz1,2,3, Edgar Bernardo4,5, Anthony Beucher1, Miguel Angel Maestro4,5, Natalia del Pozo2,3, Irene Millán2,3, Lena Haeberle6, Martin Schlensog6, Sami Alexander Safi7, Wolfram Trudo Knoefel7, Vanessa Grau4,5, Matías de Vas1, Karl B Shpargel8, Eva Vaquero9, Terry Magnuson8, Sagrario Ortega10, Irene Esposito7, Francisco X Real2,3,11 and Jorge Ferrer *,1,4,5 1Section of Epigenomics and Disease, Department of Medicine, Imperial College London, London, UK 2Epithelial Carcinogenesis Group, Spanish National Cancer Research Centre-CNIO, Madrid, Spain 3CIBERONC, Madrid, Spain 4Bioinformatics and Genomics Program, Centre for Genomic Regulation (CRG), The Barcelona Institute of Science and Technology (BIST), Barcelona, Spain 5Centro de Investigación Biomédica en Red de Diabetes y Enfermedades Metabólicas Asociadas (CIBERDEM), Barcelona, Spain 6Institute of Pathology, Heinrich-Heine University and University Hospital of Düsseldorf, Düsseldorf, Germany 7Department of Surgery, Heinrich-Heine University and University Hospital of Düsseldorf, Düsseldorf, Germany 8Department of Genetics and Lineberger Comprehensive Cancer Center, University of North Carolina at Chapel Hill, Chapel Hill, NC, USA 9CiberEHD, Institut de Malalties Digestives i Metabòliques, Hospital Clínic, IDIBAPS, Barcelona, Spain 10Transgenics Unit, Spanish National Cancer Research Centre-CNIO, Madrid, Spain 11Departament de Ciències Experimentals i de la Salut, Universitat Pompeu Fabra, Barcelona, Spain *Corresponding author. Tel: +34 933 160 197; E-mail: [email protected] The EMBO Journal (2020)39:e102808https://doi.org/10.15252/embj.2019102808 See also: S Bärthel et al. (May 2020) [The copyright line for this article was changed on 16 April 2020 after original online publication.] PDFDownload PDF of article text and main figures. Peer ReviewDownload a summary of the editorial decision process including editorial decision letters, reviewer comments and author responses to feedback. ToolsAdd to favoritesDownload CitationsTrack CitationsPermissions ShareFacebookTwitterLinked InMendeleyWechatReddit Figures & Info Abstract Defects in transcriptional regulators of pancreatic exocrine differentiation have been implicated in pancreatic tumorigenesis, but the molecular mechanisms are poorly understood. The locus encoding the transcription factor HNF1A harbors susceptibility variants for pancreatic ductal adenocarcinoma (PDAC), while KDM6A, encoding Lysine-specific demethylase 6A, carries somatic mutations in PDAC. Here, we show that pancreas-specific Hnf1a null mutant transcriptomes phenocopy those of Kdm6a mutations, and both defects synergize with KrasG12D to cause PDAC with sarcomatoid features. We combine genetic, epigenomic, and biochemical studies to show that HNF1A recruits KDM6A to genomic binding sites in pancreatic acinar cells. This remodels the acinar enhancer landscape, activates differentiated acinar cell programs, and indirectly suppresses oncogenic and epithelial–mesenchymal transition genes. We also identify a subset of non-classical PDAC samples that exhibit the HNF1A/KDM6A-deficient molecular phenotype. These findings provide direct genetic evidence that HNF1A deficiency promotes PDAC. They also connect the tumor-suppressive role of KDM6A deficiency with a cell-specific molecular mechanism that underlies PDAC subtype definition. Synopsis Whether defective transcriptional control of cell differentiation contributes to the development of pancreatic ductal adenocarcinoma (PDAC) remains poorly understood. Here, cooperation of transcription factor HNF1A with lysine-specific demethylase 6A (KDM6A/UTX) is found to activate an epithelial program in pancreatic acinar cells and to impair KrasG12D -driven tumorigenesis. HNF1A recruits KDM6A to target genes that specify acinar cell epithelial identity. HNF1A and KDM6A regulate a common transcriptional program that is defective in non-classical human PDAC. Hnf1a loss in mice cooperates with KrasG12D mutation and recapitulates Kdm6a-deficient PDAC. Video Synopsis HNF1A recruits KDM6A to activate differentiated acinar cell programs that suppress pancreatic cancer by Mark Kalisz, Jorge Ferrer and colleagues Introduction Pancreatic ductal adenocarcinoma (PDAC) is a leading cause of cancer mortality (Garrido-Laguna & Hidalgo, 2015). The incidence of PDAC is rising, yet current chemotherapies are generally ineffective (Ryan et al, 2014). Genomic analysis of PDAC has identified almost universal driver mutations in KRAS, TP53, SMAD4, and CDKN2A, among a long list of loci that show recurrent somatic mutations and structural variations (Jones et al, 2008; Biankin et al, 2012; Waddell et al, 2015; Bailey et al, 2016; Notta et al, 2016). A small subset of tumors is caused by germ-line mutations in DNA-repair genes (Waddell et al, 2015; Roberts et al, 2016), whereas GWAS have identified dozens of common variants that impact PDAC susceptibility (Childs et al, 2015; Klein et al, 2018). Genetic studies have therefore uncovered leads that promise to define molecular targets for future precision therapies. Up to 18% of PDAC tumors carry mutations in KDM6A (Waddell et al, 2015), which encodes Lysine-specific demethylase 6A (KDM6A), a component of the MLL/COMPASS transcriptional co-regulatory complex (Cho et al, 2007). KDM6A catalyzes demethylation of histone H3K27me3, a modification associated with polycomb-mediated repression (Agger et al, 2007; Lan et al, 2007; Lee et al, 2007). Most somatic pathogenic KDM6A mutations are likely to result in a loss of function, and mouse genetic studies have shown that Kdm6a and Kras mutations cooperate to promote PDAC (Mann et al, 2012; Andricovich et al, 2018). How KDM6A is recruited to its genomic targets in pancreatic cells, and the direct mechanisms through which it controls PDAC-relevant genetic programs are still poorly understood (Wang & Shilatifard, 2019). There is increasing evidence that the transcriptional regulation of differentiated pancreatic exocrine cells is tightly linked to PDAC development and subtype definition (Stanger & Hebrok, 2013; Krah et al, 2015; Diaferia et al, 2016; Martinelli et al, 2016; Cobo et al, 2018). Little is known, however, about the underlying molecular underpinnings. We have examined HNF1A, a homeodomain transcriptional regulator of liver, gut, kidney, and pancreas, which has been proposed as a candidate pancreatic tumor suppressor (Molero et al, 2012; Stanger & Hebrok, 2013; Hoskins et al, 2014; Luo et al, 2015). Human heterozygous HNF1A loss-of-function mutations cause diabetes, in part because HNF1A promotes pancreatic β-cell proliferation, and mouse Hnf1a mutations prevent the formation of large T antigen-driven β-cell tumors (Servitja et al, 2009). The function of HNF1A, however, is cell-type specific (Servitja et al, 2009), and both co-expression network analysis of PDAC samples and in vitro studies suggest that HNF1A has a tumor-suppressive function in pancreatic exocrine cells (Hoskins et al, 2014; Luo et al, 2015). Furthermore, GWAS suggest that genetic variants in the HNF1A locus predispose to PDAC (Pierce & Ahsan, 2011; Klein et al, 2018). Despite these observations, there is currently no direct mouse or human genetic evidence to incriminate HNF1A deficiency in PDAC. Here, we combine mouse genetics, transcriptomics, and genome binding studies to show that HNF1A is a major determinant for the recruitment of KDM6A to its genomic targets in acinar cells. This remodels the enhancer landscape of acinar cells and activates a broad epithelial cell transcriptional program that inhibits tumor suppressor pathways. We demonstrate that Hnf1a inactivation promotes Kras-induced PDAC, and partially phenocopies morphological features of Kdm6a-deficient PDAC. Finally, we define a subset of human tumors that exhibit HNF1A/KDM6A-deficient transcriptional programs. These findings, therefore, provide a molecular mechanism that connects the tumor-suppressive functions of KDM6A and pancreatic differentiation transcription factors. Results Hnf1a deficiency promotes Kras-induced oncogenesis To directly test the role of Hnf1a in pancreatic carcinogenesis, we created a conditional Hnf1a loss-of-function allele (Hnf1aLoxP) (Appendix Fig S1A) and used a Pdx1Cre transgene to delete Hnf1a in all pancreatic epithelial lineages (hereafter referred to as Hnf1apKO mice, Appendix Fig S1B). HNF1A is normally expressed in pancreatic acinar and endocrine cells, but not in duct cells (Nammo et al, 2002), and Hnf1apKO mice showed disrupted HNF1A expression in both acinar and endocrine cells (Appendix Fig S1C). As expected from previous studies of Hnf1a germ-line null mutants, this did not produce gross defects in pancreas organogenesis or tissue architecture (Appendix Fig S1D) although acinar cells displayed signs of markedly increased proliferation (Pontoglio et al, 1996; Lee et al, 1998; Boj et al, 2001; Molero et al, 2012) (Fig 1A). Figure 1. HNF1A deficiency leads to increased proliferation and promotes Kras-induced oncogenesis A. Representative immunofluorescence images and quantifications showing that 3-month-old Hnf1apKO mice have increased number of KI67+ (red) acinar cell nuclei co-staining with DAPI (blue) and Amylase (green). Arrows point to KI67+ acinar cells in Hnf1apKO mouse. Acinar proliferation is represented as the average of the KI67+/Amylase+ cell ratio. Quantifications were performed on 3 random fields from 3 Pdx1Cre and 3 Hnf1apKO mice. P-values are from two-tailed Student's t-test. Representative H&E stainings of pancreata from KrasG12D and Hnf1apKO;KrasG12D mice. B–D. KrasG12D and Hnf1apKO;KrasG12D mice have normal morphology at 7 days. E–J. At 21 days, Hnf1apKO;KrasG12D mice show acinar-to-ductal metaplasia (dashed encircled areas) and regions with desmoplastic reaction (asterisk), which are not observed in KrasG12D mice (E, H). K–P. At 8 weeks, KrasG12D pancreas show occasional abnormal ductal structures (dashed encircled areas in N, which is a magnification of squared dotted box in K) and Hnf1apKO;KrasG12D mice (L, M, O, P) present mucinous tubular complexes (black arrows), and more advanced PanINs with luminal budding (open arrows) including foci of spindle cell proliferation (asterisks) and incipient infiltrative growth (black dashed box area in O). Data information: Black dashed boxes in (E, F, K, L and O) indicate magnified areas in (H, G, N, M and P) respectively. Scale bars indicate 200 μm (A), 100 μm (C, E, F, K, L), 50 μm (O), and 20 μm (B, D, G, H–J, M, N, P). Download figure Download PowerPoint To determine whether Hnf1a interacts with Kras-induced carcinogenesis, we created mice with combined conditional Hnf1a and KrasG12D mutations, hereafter referred to as Hnf1apKO;KrasG12D mice (Appendix Fig S1E). In the absence of Hnf1a mutant alleles, Pdx1Cre-induced KrasG12D activation expectedly gave rise to occasional low-grade PanINs or acinar-to-ductal metaplasia (ADM) lesions by 2 months of age (Hingorani et al, 2003) (Fig 1B, E, H, K and N). Hnf1apKO;KrasG12D mice showed no lesions at 7 days of age (Fig 1C and D), yet by weaning they had already developed focal ADM and desmoplastic reactions, which became more prominent as the mice aged (Fig 1F, G, I and J and data not shown). Eight-week-old Hnf1apKO;KrasG12D mice additionally showed non-invasive atypical tubular complexes, higher-grade PanINs with luminal budding, desmoplastic reaction, and foci of spindle cell (mesenchymal) proliferation, some of which showed incipient infiltrative growth (Fig 1L, M, O and P). These findings indicate that pancreatic Hnf1a deficiency cooperates with Kras to promote sarcomatoid forms of PDAC. HNF1A activates an acinar differentiation program that inhibits oncogenic programs To understand how Hnf1a deficiency promotes pancreatic cancer, we examined the transcriptional programs controlled by Hnf1a in pancreatic exocrine cells. Genetic lineage tracing studies in mice have shown that, despite the ductal morphology of PDAC, KrasG12D-induced PDAC can originate from acinar cells that undergo ADM and PanIN, contrasting with intraductal papillary mucinous neoplasms that arise from duct cells (Kopp et al, 2012; von Figura et al, 2014). We therefore used a Ptf1aCre allele, which ensured high-efficiency recombination of Hnf1a in mouse acinar cells, and more limited recombination in endocrine cells (Ptf1aCre; Hnf1aLoxP/LoxP; hereafter referred to as Hnf1aaKO) (Fig EV1A and B). Eight-week-old Hnf1aaKO mice were normoglycemic, and like Hnf1apKO mice showed normal pancreatic histology (Fig EV1C). We profiled transcripts in pancreas from 8-week-old Hnf1aaKO mice and, despite the normal histology, found profound transcriptional changes (Fig 2A, Dataset EV1). We observed decreased expression of genes specific to differentiated acinar cells, including Ptf1a, Pla2g1b, Serpini2, and Ctrb1 (Figs 2B and EV1D), and increased expression of genes specific to pancreatic mesenchymal cells (Fig 2B, Dataset EV2). Downregulated genes were enriched in metabolic processes such as inositol phosphate turnover, amino acid metabolism, and protection against oxidative stress, whereas upregulated genes were enriched in annotations associated with the extracellular matrix (collagen formation, ECM-receptor interactions, integrin cell surface interactions) and complement activation (Fig 2C, Dataset EV2). We also observed activation of cell cycle-related pathways, cholesterol biosynthesis, and known oncogenic programs such as EMT, RAS, PI3K-AKT, STAT3, WNT, and MAPK signaling (Figs 2D and EV1E and F, Dataset EV2). Click here to expand this figure. Figure EV1. Hnf1a deletion leads to abnormal acinar cell identity and increased expression of genes related to oncogenic pathways A. Breeding strategy to generate Hnf1aaKO and Ptf1aCre;Hnf1a+/+ control mice using Ptf1aCre and Hnf1aLoxP alleles. B. Ptf1aCre deletes HNF1A efficiently in acinar cells but to a lesser extent in islets of Langerhans. HNF1A IHC and hematoxylin staining in pancreas of control and Hnf1aaKO mice. HNF1A is expressed in acinar and islet cells, but not in ductal cells in normal pancreas (left). HNF1A expression is depleted in acinar cells but largely not in islets in Hnf1aaKO pancreas (right). The squared dotted boxes (top) indicate magnified areas (bottom). Arrows point at ducts, arrow head at HNF1A-positive acinar cell, and open arrow head at HNF1A positive islet cell. The dotted encircled areas indicate islets of Langerhans. Scale bar (top) 300 μm (bottom) 50 μm. C. H&E stainings in pancreas of control (left) and Hnf1aKO mice (right) showing unaltered pancreatic morphology. Scale bar 300 μm. D. Expression of acinar differentiation genes in pancreas from Hnf1aaKO and controls, depicted as box plots with median values and IQR of TPM values. Whiskers extend to highest and lowest data points within 1.5× IQR outside box limits. P-values were determined by two-tailed Student's t-test and n = 3 replicates per condition. E. GSEA showing increased expression of oncogenic pathways in Hnf1aaKO pancreas. F. Western blots (top) and quantifications (bottom) showing increased phospho-p42 levels in Hnf1aaKO pancreas. Quantification of signal intensities of phospho-p44/p42 normalized to total-p44/p42 levels. Data are shown as dots with mean and error bars ± SD. P-values were determined by two-tailed Student's t-test. G. Distribution of pancreatic HNF1A binding sites in annotated genomic regions. H. Venn diagrams illustrating that HNF1A-bound regions are enriched in regions of active promoters and enhancers. P-values and odds ratios were calculated by Fisher's exact test. I. Enrichment of known HNF1 motifs in the top 500 most significant HNF1A-bound ChIP-seq regions and percentage of regions containing each motif. The “union” is the percentage of regions with at least one motif sequence occurrence. Enrichment P-values are calculated using the one-tailed binomial test. J. Genome browser track for Fn1 and Timp1 genes showing upregulated expression in Hnf1aaKO and Kdm6apKO pancreas, and absence of HNF1A or KDM6A binding in adjacent regions. Plots show TPM values normalized to Hprt with mean and error bars ± SD. P-values were determined by two-tailed Student's t-test. Download figure Download PowerPoint Figure 2. HNF1A regulates gene programs essential to acinar cell identity, metabolism, and proliferation A. Fold change (FC) in transcripts in Hnf1aaKO versus control pancreas, plotted against significance (−Log10 q; genes significant at q < 0.05 are shown as colored dots above the horizontal line). B. GSEA showing that genes specific to differentiated acinar cells were downregulated in Hnf1aaKO pancreas, but not genes specific to islets or duct cells. Upregulated genes were enriched in genes specific to mesenchymal cells. Lineage-enriched genes were obtained from Muraro et al (2016). C. Top functional annotations for differentially expressed genes in Hnf1aaKO pancreas. D. GSEA revealed that Hnf1aaKO pancreas showed increased transcripts involved in oncogenic pathways such as EMT, MAPK, KRAS, PI3K-AKT. E. HNF1A promotes transcriptional activation of direct target genes. Left: HNF1A-bound genes were enriched among genes that showed downregulation in Hnf1a mutants, but not among upregulated genes. P-values and odds ratios (O.R.) calculated by Fisher's exact test. Right: Venn diagrams showing overlap of HNF1A-bound genes with genes that were downregulated and upregulated in Hnf1a mutant pancreas. Download figure Download PowerPoint To assess which of these transcriptional changes reflects a direct function of HNF1A in acinar cells, we profiled genome-wide binding sites of HNF1A in adult pancreas (Fig EV1G), as well as H3K27 acetylation to mark active enhancers and promoters (Fig EV1H). HNF1A-bound genomic regions had canonical HNF1 recognition sequences in 405 out of the top 500 most significant binding sites (81%), confirming the specificity of the assay (Fig EV1I), and they were expectedly enriched in enhancers and promoters (Fig EV1H). HNF1A binding was specifically enriched among genes that showed downregulation in Hnf1aaKO pancreas (odds ratio = 3.6 relative to all other genes, P = 10−22, Fig 2E). This was consistent with HNF1A acting as an essential positive regulator of some of the genes to which it binds. HNF1A did not, however, show a higher number of binding near genes that were upregulated in Hnf1aaKO pancreas, compared with active genes that did not show altered regulation (odds ratio = 0.4, P = N.S.; Figs 2E and EV1J). These studies, therefore, uncovered direct HNF1A-dependent genetic programs. They show that the function of HNF1A in pancreatic acinar cells entails direct transcriptional activation of a broad differentiated cell program that controls metabolic functions. They also revealed that HNF1A suppresses growth-promoting pathways and that, given the absence of enriched HNF1A binding to upregulated genes, this is largely mediated through indirect regulatory mechanisms. HNF1A-dependent programs in human acinar cells We explored the relevance of mouse pancreatic exocrine HNF1A-dependent programs to human acinar cells. Given the lack of human acinar cell models to perform genetic manipulations, we examined transcriptomes from 328 human pancreas samples from the Genotype-Tissue Expression (GTEx) project (Consortium et al, 2017). We defined GTEx samples with highest and lowest deciles of HNF1A mRNA expression (n = 33 per group) and asked if human orthologs of genes that were deregulated genes in Hnf1aaKO pancreas showed concordant changes. We observed that Hnf1aaKO up- and downregulated genes showed consistent up- and downregulation in HNF1A-low versus HNF1A-high human pancreas (median Log2 fold-difference [IQR]: upregulated genes 0.44 [0.05–0.81]; downregulated genes −0.16 [−0.38 to 0.05]; control genes 0.03 [−0.2 to 0.36]; Kruskal–Wallis P < 10−4) (Fig 3A). Accordingly, 70 and 75% of Hnf1aaKO up- and downregulated genes showed differential expression in the same direction at nominal P < 0.05 in HNF1A-low versus HNF1A-high human pancreas, respectively. These observations suggested that HNF1A-dependent programs are largely conserved in mouse and human pancreatic cells. Figure 3. Human non-classical and KDM6A-deficient PDAC exhibit HNF1A-deficient phenotypes A. Human orthologs of genes that were up- and downregulated in Hnf1aaKO pancreas were also up- and downregulated in human pancreas with low versus high HNF1A expression (lowest versus highest expression deciles, respectively). A random list of 717 genes controlled for similar expression levels was used for comparison. Violin plots include median a
更多查看译文
关键词
pancreatic cancer,hnf1a,kdm6a,acinar cell programs
AI 理解论文
溯源树
样例
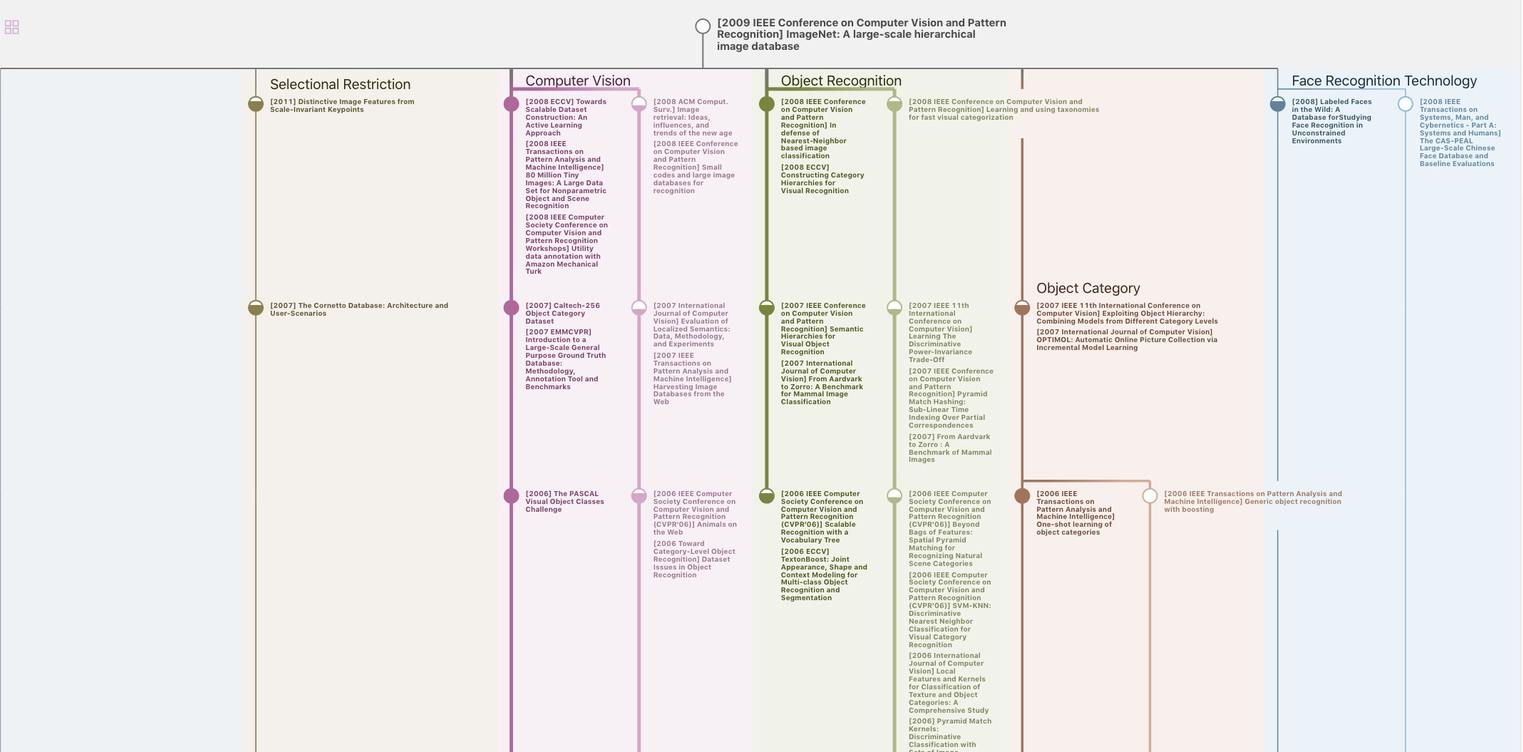
生成溯源树,研究论文发展脉络
Chat Paper
正在生成论文摘要