Biodegradation of waste PET
EMBO Reports(2019)
摘要
Science & Society24 October 2019free access Biodegradation of waste PET A sustainable solution for dealing with plastic pollution Kazumi Hiraga Department of Applied Biology, Faculty of Textile Science, Kyoto Institute of Technology, Kyoto, Japan Search for more papers by this author Ikuo Taniguchi International Institute for Carbon-Neutral Energy Research (I2CNER), Kyushu University, Fukuoka, Japan Search for more papers by this author Shousuke Yoshida Division for Research Strategy, Institute for Research Initiatives, Nara Institute of Science and Technology, Nara, Japan Search for more papers by this author Yoshiharu Kimura Center for Fiber and Textile Science, Kyoto Institute of Technology, Kyoto, Japan Search for more papers by this author Kohei Oda [email protected] Department of Applied Biology, Faculty of Textile Science, Kyoto Institute of Technology, Kyoto, Japan Search for more papers by this author Kazumi Hiraga Department of Applied Biology, Faculty of Textile Science, Kyoto Institute of Technology, Kyoto, Japan Search for more papers by this author Ikuo Taniguchi International Institute for Carbon-Neutral Energy Research (I2CNER), Kyushu University, Fukuoka, Japan Search for more papers by this author Shousuke Yoshida Division for Research Strategy, Institute for Research Initiatives, Nara Institute of Science and Technology, Nara, Japan Search for more papers by this author Yoshiharu Kimura Center for Fiber and Textile Science, Kyoto Institute of Technology, Kyoto, Japan Search for more papers by this author Kohei Oda [email protected] Department of Applied Biology, Faculty of Textile Science, Kyoto Institute of Technology, Kyoto, Japan Search for more papers by this author Author Information Kazumi Hiraga1, Ikuo Taniguchi2, Shousuke Yoshida3, Yoshiharu Kimura4 and Kohei Oda5 1Department of Applied Biology, Faculty of Textile Science, Kyoto Institute of Technology, Kyoto, Japan 2International Institute for Carbon-Neutral Energy Research (I2CNER), Kyushu University, Fukuoka, Japan 3Division for Research Strategy, Institute for Research Initiatives, Nara Institute of Science and Technology, Nara, Japan 4Center for Fiber and Textile Science, Kyoto Institute of Technology, Kyoto, Japan 5Department of Applied Biology, Faculty of Textile Science, Kyoto Institute of Technology, Kyoto, Japan EMBO Rep (2019)20:e49365https://doi.org/10.15252/embr.201949365 Correction(s) for this article Biodegradation of waste PET05 February 2020 PDFDownload PDF of article text and main figures.AM PDF ToolsAdd to favoritesDownload CitationsTrack CitationsPermissions ShareFacebookTwitterLinked InMendeleyWechatReddit Figures & Info With the discovery of bakelite in 1907 by Leo Baekeland began the age of plastics. Since a wide variety of synthetic polymers have been developed and have become ubiquitous and indispensable not only in human life but also, unfortunately, in the environment too. In 2015, 5,000 Mt of plastic waste had been accumulated in landfills and elsewhere and that amount may well increase to 12,000 Mt by 2050 1. Most plastics—notably polyethylene (PE), polypropylene (PP), polystyrene (PS), polyvinylchloride (PVC), and poly(ethylene terephthalate) (PET)—are made from fossil hydrocarbons, a non-renewable resource. Despite these obvious problems, the production volume of these plastics was 380 Mt per year in 2015 1, a level that is expected to increase during the next decades. The growing awareness of the environmental impact of plastics has therefore inspired the search for new technologies and other solutions not only to deal with the increasing amount of plastic waste in the environment but also to decrease the dependency on crude oil as the source of plastic monomers and other precursor chemicals. The growing awareness of the environmental impact of plastics has therefore inspired the search for new technologies and other solutions… The largest market for plastics is packaging, where plastic materials are designed for a short-time single use. Another major market is synthetic fibers and clothing that, during production and washing, shed microfibers that are released into waterways. Owing to their non-degradable nature, these fibers end up in the oceans or on coastlines along with plastic bottles, packages, and other wastes. PET and its recycling Poly(ethylene terephthalate) is synthesized by the polycondensation of terephthalic acid (TPA) and ethylene glycol (EG), or by transesterification of dimethyl terephthalate and EG. Since its development by DuPont in the mid-1940s, it has become one of the most abundant mass-produced thermoplastics. Owing to its excellent mechanical properties, thermal stability, impermeability for liquids and gases, and transparency, it is mostly used for textile fibers, beverage bottles, and food containers. However, as PET is a very stable polymer and resistant to hydrolytic or enzymatic degradation, it has become a major component of plastic waste found in the environment, along with PE, PP, and PS. Collection programs for plastic waste have been successfully implemented in various countries. In addition to producing heat or electricity by incinerating discarded plastic containers, collected PET waste is cut into flakes and reprocessed to films, sheets, or textile fibers, a process called “material recycling”. However, recycling requires heating of the plastic, which leads to thermal degradation of the polymer chains, and the loss of their mechanical properties; thus, beverage bottles can no longer be reprocessed to beverage bottles. In addition, reprocessed materials through thermal degradation of polymer chains cannot be further recycled and are incinerated, thereby generating CO2 emissions. Further studies led to the discovery of a bacterial species that is able to metabolize amorphous PET without any assistance from other organisms in the consortium. In contrast, a “chemical recycling” process decomposes PET to its constituent monomers that can be repolymerized to synthesize plastics with the original material properties. Unfortunately, reprocessed resin is more expensive than monomers from fossil fuels, which has made chemical recycling economically unattractive. What is needed is an effective and economical alternative to recycle PET waste by either breaking it down into its monomers for repolymerization or other compounds that can be reused for different purposes. Here, we discuss the biodegradation of waste PET as a potential solution for reducing plastic pollution in a sustainable way. Biodegradation of waste PET Poly(ethylene terephthalate) monomers are linked by ester bonds, which can be hydrolyzed by many hydrolytic enzymes found in nature. Theoretically, this makes PET more amenable to natural degradation than the polyolefins, as there are no known enzymes that could directly break their C–C bonds. Yet, although many PET hydrolytic enzymes (PHEs) have been identified, their capacity to degrade PET and use it as a carbon source for the microbial organism had not been demonstrated. A major reason for the poor biodegradability of PE and other polyolefins is minimally reactive C–C bonds in the backbones. Other factors, such as flexibility of the polymer chain, its crystallinity, and hydrophobicity of the surface, also limit enzymatic degradation. We screened for microorganisms that degrade PET through cultivation of environmental samples including PET debris. They were also expected to possess active PHEs. We eventually found a unique microbial consortium, named No. 46, in a landfill. This consortium is able to grow on low-crystallinity PET film 2; it assembles on the film and utilizes PET as a major carbon and energy source, degrading it into CO2 and water. Further studies led to the discovery of a bacterial species that is able to metabolize amorphous PET without any assistance from other organisms in the consortium. This strain, a gram-negative, aerobic, rod-shaped bacterium, was found to be a new species of the genus Ideonella, and it was named Ideonella sakaiensis 201-F6 3. With a rapid increase in the number of microbial genomes and metagenomes being sequenced, it is likely that potential PET metabolic enzymes might be discovered in the near future. The sequencing of the I. sakaiensis genome enabled the identification of an enzyme with PET-hydrolyzing properties. It produces crater-like pitting on the surface of an amorphous PET film and releases the hydrolysis products into the aqueous environment 2. Comparison of this enzyme and other known PHEs revealed that the I. sakaiensis enzyme has the highest catalytic preference for PET at ambient temperature 2; we therefore designated it a PET hydrolase (PETase). Phylogenetic tree analysis of PHEs shows that these enzymes appear in both eukaryotes and bacteria 4. The bacterial domain that expresses PHEs is further divided into two major branches belonging to the phyla Actinobacteria and Proteobacteria. Through structure determination 5 and comparison of the PETase from I. sakaiensis, a proteobacterial strain, with the Thermobifida fusca cutinase, an actinobacterial PHE, researchers identified several unique features of PETase. The PET binding cleft is broader in PETase than in the T. fusca cutinase and provides more space to accommodate PET as a substrate 5; two unique cysteine residues in PETase form a disulfide bond at the vicinity of the active site which controls the active site flexibility of mesophilic PETase. These characteristics are likely to contribute to efficient PET hydrolysis. Recovering plastic monomers Ideonella sakaiensis possesses another enzyme involved in PET metabolism, which has been classified as a member of the tannase family. This enzyme hydrolyzes (2-hydroxyethyl) terephthalic acid (MHET), the main PET hydrolysis product of PETase, into TPA and EG 2. While it is highly active and specific to MHET, it has insignificant hydrolytic effect on bis-(2-hydroxyethyl) TPA (BHET) and several other substrates; we therefore designated this enzyme as MHET hydrolase (MHETase). The crystal structure showed that it is composed of an α/β-hydrolase domain and a lid domain 6. While the α/β-hydrolase domain includes amino acid residues essential for catalysis, the lid domain provides substrate specificity. The structural and biochemical analyses for MHETase identified that MHET binding to the catalytic pocket induces a structural change that closes the active site, and that six amino acid residues in the lid domain have in direct contact with the substrate, which results in stricter substrate recognition and tighter binding. The discovery of Ideonella sakaiensis and its capacity to assimilate PET […] could provide a potential solution for bio-recycling of PET. PETase and MHETase catalyze similar reactions in different locations. Extracellular PETase hydrolyzes PET into MHET, which is transported into the periplasmic space of I. sakaiensis where outer membrane-anchored MHETase hydrolyzes MHET into TPA and EG 2, 4. This combination and arrangement of enzymes for metabolizing PET has so far not been found in any other organisms with fully sequenced genomes. However, there might well exist similar systems with even more potential for PET degradation in other microorganisms. With a rapid increase in the number of microbial genomes and metagenomes being sequenced, it is likely that potential PET metabolic enzymes might be discovered in the near future. Potential applications for PETase The discovery of I. sakaiensis and its capacity to assimilate PET through hydrolysis, followed by downstream metabolism of its monomers, could provide a potential solution for biological recycling of PET either using I. sakaiensis directly or its PET-hydrolyzing enzymes through metabolic engineering of other organisms. We think that further biodegradation of PET can become an important innovative technology for a circular economy in the near future and for meeting Sustainable Development Goals. Ideonella sakaiensis and other organisms and their hydrolyzing enzymes 2 would be central for developing various potential applications (Fig 1) 4. Figure 1.A circular economy based on PET biodegradation and conversion. Download figure Download PowerPoint Alternatively, I. sakaiensis could also be adapted to recapture monomers for plastic synthesis. One approach would use microbial consortium No. 46, which is composed of bacteria, yeast-like eukaryota, and other protozoa. No. 46's PET degradation activity remains stable even after repeated subculture, which would allow its use either in the environment or in the specialized facilities to degrade marine and terrestrial PET waste and microplastics, in particular as the biodegradation processes require no special culturing equipment, medium or further supply of energy. The consortium could also be used for bioremediation of liquid waste containing oligomers and monomers generated during alkali treatment of PET fibers in the textile industry. CO2 generated from by degradation of PET could be used for growing plants and algae so as to mitigate CO2 emissions. Such a combination of waste PET degradation and CO2 fixation would create a circular system to recapture monomers and energy that goes beyond the conventional chemical recycling process. While consortium No. 46 includes PET-degrading I. sakaiensis 201-F6 2, 3, other bacteria with specialized functions involved in PET degradation were isolated and characterized. Initially, Bacillus megaterium forms a biofilm on the PET film. Within this biofilm, Rhizobium sp. degrades PET into BHET, which is further degraded into TPA and EG by Pseudomonas sp. TPA and EG are eventually assimilated by Pigmentiphaga sp. and Mycobacterium sp., respectively. Genome sequences of these bacteria would allow us to identify the genes that contribute to the degradation of PET and oligomers (BHET and MHET) and to engineer microorganisms with improved PET-degrading abilities. Based on the available 3D structures of PETase, it should also be possible to improve the thermal stability and activity of PETase and MHETase through protein engineering, whereas the addition of surfactants will help to accelerate PET degradation. Elucidation of the molecular adhesion mechanism of I. sakaiensis 201-F6 to PET film, including cell appendages and other structural components, will also be necessary to improve the efficiency of PET degradation, and to preserve the highly concentrated micro-environment of PET-degrading enzymes with their serial and efficient actions. Moreover, the isolation of marine microorganisms that express or secrete salt-tolerant PET-degrading enzymes could provide an efficient approach to degrade plastic waste and microplastics directly in the oceans or on coastlines. Alternatively, I. sakaiensis could also be adapted to recapture monomers for plastic synthesis. Here, it would be necessary to disrupt genes in the downstream monomer degradation pathway, for instance by interrupting TPA degradation. It would also allow bioconversion of PET waste to other compounds, such as conversion of TPA to protocatechuic acid (PCA) with simultaneous disruption of the PCA metabolic pathway. In the future, genome sequence data and protein structure data from many organisms, metabolic engineering, and synthetic biology could be used to create synthetic organisms—bacteria or microalgae—as cell factories for producing specific, high-value compounds with high titer and yield directly from PET or other plastics. In summary, we can envision various applications of either consortium No. 46, I. sakaiensis 201-F6, or newly engineered microorganisms with plastic-hydrolyzing properties. These could be used to digest waste PET collected from the oceans, coastline into CO2, and water in waste treatment facilities. Alternatively, PET-degrading microorganisms and their enzymes can be used to break down waste PET into its constituent units such as TPA and MHET for recovery and “bio-recycling”. This could be easily modified to convert these monomers into other chemicals: TPA can be converted to PCA and adipic acid, while EG can be used for producing ethanol or glycolic acid. EG could also serve as a substrate for producing bioplastics such as poly-hydroxyalkanoates via engineered microorganisms. A somewhat different application would be the surface treatment of PET fibers for improved texture and staining in the textile industry which would also prevent the leaching of microfibers into the environment. Finally, PETase could be applied for the biodegradation of poly(ethylene furanoate) (PEF) 7, which is a PET alternative and a 100% bio-based biodegradable plastic material for bioeconomy. Biodegradation of PET in a bioeconomy Our discovery of I. sakaiensis shows that PET-degrading bacteria exist in nature, even if PET hydrolase occurs only rarely in environmental microbiota 5. In the future, it will be necessary to isolate and study more microorganisms and enzymes that can degrade PET at higher temperature. With the PET-degrading enzyme from I. sakaiensis 201-F6 as the so far best-studied model, it would allow the design of thermostable PETase based on 3D structures. Moreover, the use of surfactants can enhance PET degradation at least by a factor of 120 8. In addition, isolation and analysis of other PET-degrading organisms and enzymes from high-salt environment are also important for the biodegradation of waste PET directly in the ocean, coastline, and estuaries, to address the problems caused by the spread of microplastics. The discovery of PET biodegradation has raised the possibility of finding other biodegradation pathways that would be necessary for dealing with these plastic wastes. All of this could inspire a new, sustainable bio-industry that uses biochemical syntheses and conversions, along with new environmental technologies. It would also herald a new “bioeconomy” based on recycled and renewable resources instead of fossil resources 9. Ideonella sakaiensis has great potential as a key species for PET degradation in this circular economy. Plastics, or more generally, polymeric materials, can be classified into four categories: bio-based polymers that are (i) biodegradable or (ii) not and oil-based polymers (iii) with and (iv) without biodegradability (Fig 2). The recent dispute about plastic waste in marine environments has prompted us to divide the biodegradable polymers further by whether they can be degraded in sea water or not. In addition, one could consider whether polymers in (ii) and (iv) can be microbially assimilated or not. Figure 2. Classification of polymeric materials in terms of bio-based and biodegradable nature. Download figure Download PowerPoint Whether a polymeric material is biodegradable or not is determined by tests that are strictly standardized by the International Organization for Standardization (ISO) or by related organizations of individual countries. Generally, a polymer sample is subjected to a biodegradation test either with compost or with activated sludge, and the degradation time is compared with a biodegradable reference sample—for instance, cellulose. For a polymer to be categorized as “biodegradable”, more than 60–70% of the sample must be degraded within 6 months. PET cannot meet these conditions and is accordingly categorized as a non-biodegradable polymer. Thus, the discovery of microbial assimilation of PET is very important because it suggests the possibility that waste PET in the environment can be biologically eliminated, even if it takes a long time. Plastic pollution As discussed above, the use of biodegradable polymers can help to prevent the accumulation of discarded plastics in the environment. However, this may not be sufficient to deal with the plastic waste that is already in the environment. For solving the problems of microplastics and marine and terrestrial contamination, bioremediation may be the only solution by improving the decontamination function of nature 10. A vast number of organisms exhibit formidable capabilities to metabolize environmental contaminants, or microorganisms evolve so as to degrade non-natural substances. Indeed, PET-degrading microorganisms may have just recently evolved to use PET waste as an abundant carbon and energy source. Given the density of PET (1.27 g/cm3), solid plastic in the oceans should quickly accumulate on the seabed, while empty PET bottles and expanded PET containers would be able to float on the sea surface. It takes a long time until these solid plastics are disintegrated. Most of the microplastic pollution is caused by surface fragmentation: PET textiles, for instance, constantly shed fibers during washing or physical and chemical treatments that eventually end up in the oceans and sink to the bottom sea. Since the PET-degrading I. sakaiensis works at ambient temperature, it could become an impart part for any solutions to degrade plastics in marine environments. Furthermore, microbial consortia created on amorphous PET films can be utilized more effectively to support their PET-degrading microorganism for bioremediation. As noted above, six polymers (PE, PP, PVC, PET, polyurethane (PUR), and PS)—all of which are petroleum-based and non-degradable—comprise more than 90% of the global total production of polymeric materials. The overall ratio of PET is around 17%, including the PET present in more than 70% of all fibers and textiles. Even if bioremediation of PET-based pollutants becomes possible, it still leaves the challenge whether polyethylene (PE) and polymers can be degraded in the natural environment. The discovery of PET biodegradation has raised the possibility of finding other biodegradation pathways that would be necessary for dealing with these plastic wastes. All of this will require intimate cooperation of many sectors and different disciplines not only to change industrial production toward bio-based systems but also to address social, political, and other non-scientific problems and challenges. Even the easiest application, the biodegradation of PET materials, requires the expertise of biology and material sciences. Cheap and easy methods to produce bio-based and biodegradable polymers must be developed to substitute the conventional oil-based, non-degradable plastics, reduce CO2 emissions, and solve the plastic waste problems. This challenge will again require cooperation of researchers with different expertise from the natural sciences, economic sciences, and social sciences. Furthermore, a global view of the problem is necessary both for addressing global problems and for managing regional issues and different environmental conditions. Biology, through the discovery of microorganisms that can degrade plastics and other non-natural chemicals, offers solutions to many pressing environmental problems. It is up to scientists from all disciplines, however, to make these works. Further reading Plastic pollution and biodegradation do Sul JAI, Costa MF (2014) The present and future of microplastic pollution in the marine environment. Environ Pollut 185: 352–364 Shah AA, Hasan F, Hameed A, Ahmed S (2008) Biological degradation of plastics: a comprehensive review. Biotechnol Adv 26: 246–265 Wei R, Zimmermann W (2017) Biocatalysis as a green route for recycling the recalcitrant plastic polyethylene terephthalate. Microb Biotechnol 10: 1302–1307 Funabashi M, Ninomiya F, Kunioka M (2009) Biodegradability evaluation of polymers by ISO 14855-2. Int J Mol Sci 10: 3635–3654 Mohan N, Usha R (2018) Bio-augmentation – effective method of treating plastic waste – a field Study, J Pure Appl Microbiol 12: 1641–1646 Microbial hydrolases that degrade PET Müller RJ, Schrader H, Profe J, Dresler K, Deckwer WD (2005) Enzymatic degradation of poly(ethylene terephthalate): rapid hydrolyse using a hydrolase from T. fusca. Macromol Rapid Comm 26: 1400–1405 Han X, Liu W, Huang JW, Ma J, Zheng Y, Ko TP, Xu L, Cheng YS, Chen CC, Guo RT (2017) Structural insight into catalytic mechanism of PET hydrolase. Nat Commun 8: 2106 Fecker T, Galaz-Davison P, Engelberger F, Narui Y, Sotomayor M, Parra LP, Ramirez-Sarmiento CA (2018) Active site flexibility as a hallmark for efficient PET degradation by I. sakaiensis PETase. Biophys J 114: 1302–1312 Liu B, He L, Wang L, Li T, Li C, Liu H, Luo Y, Bao R (2018) Protein crystallography and site-direct mutagenesis analysis of the poly(ethylene terephthalate) hydrolase PETase from Ideonella sakaiensis. Chembiochem 19: 1471–1475 Danso D, Schmeisser C, Chow J, Zimmermann W, Wei R, Leggewie C, Li X, Hazen T, Streit WR (2018) New insights into the function and global distribution of polyethylene terephthalate (PET)-degrading bacteria and enzymes in marine and terrestrial metagenomes. Appl Environ Microbiol 84: e02773-02717 References 1. Geyer R, Jambeck JR, Law KL (2017) Production, use, and fate of all plastics ever made. Sci Adv 3: e1700782CrossrefPubMedWeb of Science®Google Scholar 2. Yoshida S, Hiraga K, Takehana T, Taniguchi I, Yamaji H, Maeda Y, Toyohara K, Miyamoto K, Kimura Y, Oda K (2016) A bacterium that degrades and assimilates poly(ethylene terephthalate). Science 351: 1196–1199CrossrefCASPubMedWeb of Science®Google Scholar 3. Tanasupawat S, Takehana T, Yoshida S, Hiraga K, Oda K (2016) Ideonella sakaiensis sp. nov., isolated from a microbial consortium that degrades PET. Int J Syst Evol Microbiol 66: 2813–2818CrossrefCASPubMedWeb of Science®Google Scholar 4. Taniguchi I, Yoshida S, Hiraga K, Miyamoto K, Kimura Y, Oda K (2019) Biodegradation of PET: current status and application aspects. ACS Catal 9: 4089–4105CrossrefCASWeb of Science®Google Scholar 5. Joo S, Cho IJ, Seo H, Son HF, Sagong HY, Shin TJ, Choi SY, Lee SY, Kim KJ (2018) Structural insight into molecular mechanism of poly(ethylene terephthalate) degradation. Nat Commun 9: 382CrossrefPubMedWeb of Science®Google Scholar 6. Palm GJ, Reisky L, Bottcher D, Muller H, Michels EAP, Walczak MC, Berndt L, Weiss MS, Bornscheuer UT, Weber G (2019) Structure of the plastic-degrading Ideonella sakaiensis MHETase bound to a substrate. Nat Commun 10: 1717CrossrefPubMedWeb of Science®Google Scholar 7. Austin HP, Allen MD, Donohoe BS, Rorrer NA, Kearns FL, Silveira RL, Pollard BC, Dominick G, Duman R, El Omari K et al (2018) Characterization and engineering of a plastic-degrading aromatic polyesterase. Proc Natl Acad Sci USA 115: E4350–E4357CrossrefCASPubMedWeb of Science®Google Scholar 8. Furukawa M, Kawakami N, Oda K, Miyamoto K (2018) Acceleration of enzymatic degradation of poly(ethylene terephthalate) by surface coating with anionic surfactants. Chem Sus Chem 11: 4018–4025Wiley Online LibraryCASWeb of Science®Google Scholar 9. Narancic T, O'Connor KE (2019) Plastic waste as a global challenge: are biodegradable plastics the answer to the plastic waste problem? Microbiology 165: 129–137CrossrefCASPubMedWeb of Science®Google Scholar 10. Aziz SS, Malik MF, Butt I, Fatima SI, Hanif H (2018) Bioremediation of environmental waste: a review. UW J Sci Tech 2: 35–42Google Scholar Previous ArticleNext Article Read MoreAbout the coverClose modalView large imageVolume 20,Issue 11,05 November 2019Caption: Interpretation of Rembrandt van Rijn's iconic oil painting The Anatomy Lesson of Dr. Nicolaes Tulp (1632). Instead of a human subject, a neuron's axon is being dissected under the watchful eye of modern‐day molecular neurobiologists. 2019 marks the 350 th anniversary of Rembrandt's passing and is therefore declared Year of Rembrandt. In the accompanying article, the authors dissect the role and regulation of the actin‐microtubule crosslinking protein Gas2L1 in axon maturation. By Dieudonnée van de Willige, Jessica J.A. Hummel, Celine Alkemade, Gijsje H. Koenderink, Casper C. Hoogenraad, Anna Akhmanova and colleagues: Cytolinker Gas2L1 regulates axon morphology through microtubule‐modulated actin stabilization. Cover concept by Dieudonnée van de Willige. Cover image by Jasper Landman (BobFzbl Photography, www.bobfzbl.com). Volume 20Issue 115 November 2019In this issue FiguresReferencesRelatedDetailsLoading ...
更多查看译文
关键词
waste
AI 理解论文
溯源树
样例
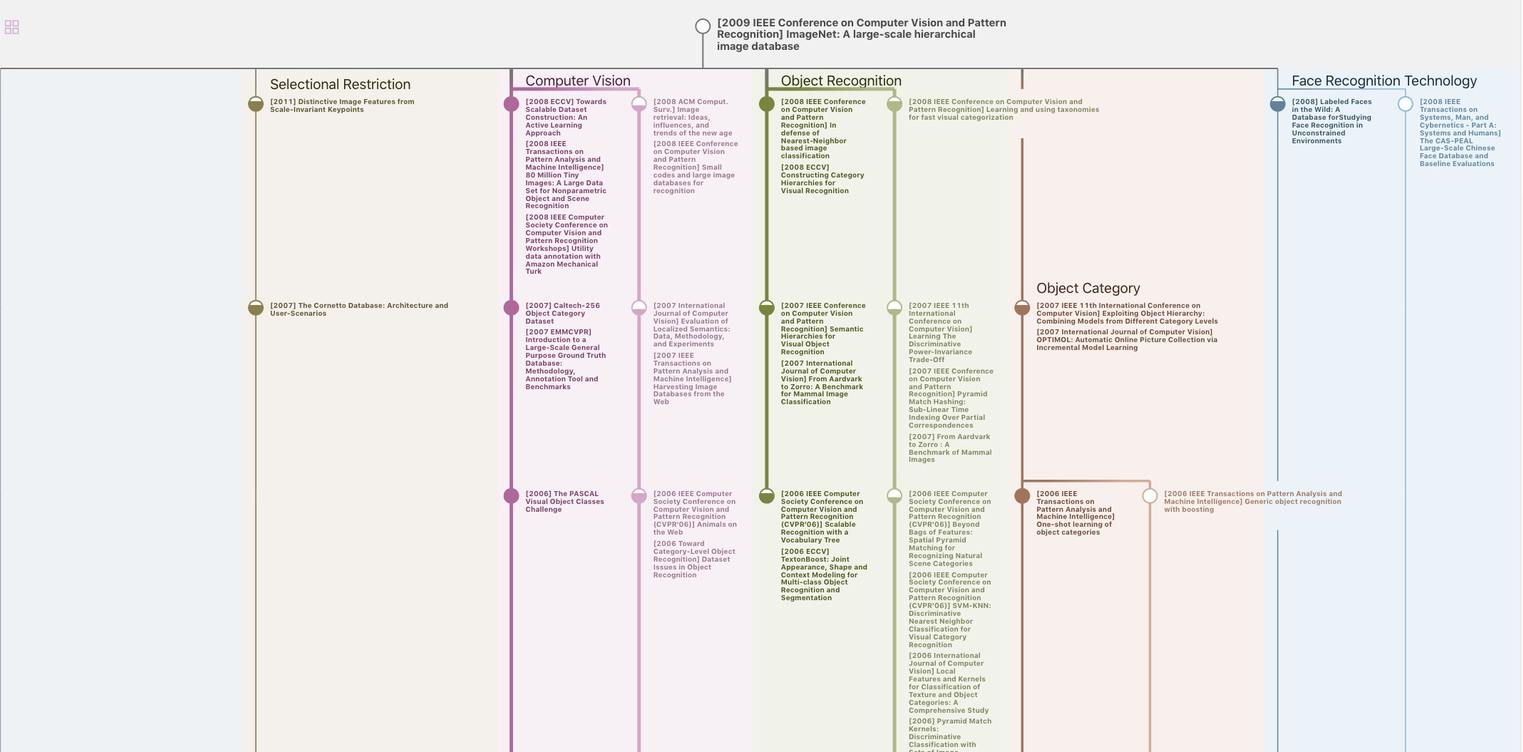
生成溯源树,研究论文发展脉络
Chat Paper
正在生成论文摘要