Genetic and morphological data suggest a southeast Australian type locality for Tursiops cymodoce (Gray, 1846)
MARINE MAMMAL SCIENCE(2024)
摘要
The increase in ocean-going scientific expeditions at the end of the 1800s provided an opportunity to increase the sightings and acquisition of cetacean specimens for museum collections, leading to a boom in the description of new species. As recently highlighted in an extensive review of the nominal species of small cetaceans (Jefferson, 2021), many of these species' descriptions were based on single specimens and did not take into consideration geographic variation. Further, some were named based solely on field observations (i.e., without a specimen in hand) or without identification of the geographic area of origin, and therefore were not recognized as valid species. Due to this potential inaccuracy in details provided during the original descriptions of some nominal species, nomenclatural confusion can be a significant issue in taxonomic endeavors. With the advent of genetics and the integration of different lines of evidence (e.g., genetics and morphology), recent taxonomic revisions of marine mammals are revealing the presence of new, and resurrecting old, taxonomic units (e.g., Rosel et al., 2021; Yamada et al., 2019). The recent rise in the number of described and recognized taxonomic units, and potentially more to come, makes it critically important to ensure all nomenclatures are accurate (Jefferson, 2021). In this study, we aimed to contribute to nomenclature stability in the genus Tursiops by investigating the provenance of a nominal species of bottlenose dolphin, Tursiops cymodoce (Gray, 1846). This species is not currently recognized (it has been synonymized with T. truncatus), but its name has been a source of potential nomenclatural confusion in the past. It was considered as a potential representative of a recently recognized coastal subspecies of Tursiops truncatus in the western South Atlantic (wSA) due to the description of its geographic area of origin (Costa et al., 2016; Wang et al., 2021). However, there were inconsistencies in the reporting of its geographic origin, and although it was rejected as the type specimen of the wSA coastal subspecies (T. t. gephyreus; see Wang et al., 2021), the lack of clarification on its provenance can potentially lead to future inaccurate nomenclatural acts, especially in a genus where descriptions of new and old taxonomic units are recently on the rise. The nominal species T. cymodoce was described based on the skull of a young animal, archived in the marine mammal collection of the Natural History Museum in London (under the accession number NHMUK GERM.355a). John E. Gray, Keeper of Zoology (1840–1874) in the natural history departments at the British Museum (these departments later became the Natural History Museum, London) originally named it Delphinus cymodoce and later renamed it to Tursio cymodoce (hereafter Tursiops cymodoce). The holotype specimen was collected from an unknown locality (Gray, 1846, 1866) during the voyage of H.M.S. Erebus and H.M.S. Terror to the Southern Hemisphere in 1839–1843, as described in the records of Richardson and Gray (1846). In a later publication, Gray (1871), without further details other than a reference to the Museum of Buenos Aires, added a type locality for the holotype: the Uruguay River, which drains into the estuary of the La Plata River, between Uruguay and Argentina in the wSA. Although H.M.S. Erebus and H.M.S. Terror had traveled through the wSA, the specific region of the La Plata River (and Uruguay River) was never in the route of their voyage (Palin, 2018). More specifically, the expedition did visit a variety of pelagic islands of the Atlantic, including the Island of Trinidad off Brazil in 1839 and the Falkland (Malvinas) Islands in 1842. The only place in the South American mainland that was visited was Rio de Janeiro (Brazil) in 1843 during a roundabout return from South Africa to Great Britain (Palin, 2018). Thus, these locations in wSA where the expedition passed through were more than 1,300 nautical miles away from the type locality in South America that Gray later assigned to the T. cymodoce holotype skull. Due to the incongruency between the later description of the type locality as Uruguay River (Gray, 1871) and the lack of this location in the route of H.M.S. Erebus and H.M.S. Terror, one can wonder: was the type specimen not collected during the voyage, despite being described and illustrated in the summary of the voyage (Gray, 1846), or is Gray's later description of the type locality incorrect? Costa et al. (2016) hypothesized that Gray's 1871 addition of the Uruguay River as the type locality for the holotype T. cymodoce may have simply followed from Burmeister's (1867) work wherein he named two skulls deposited in the Museum of Buenos Aires as “Delphinus (Tursio) Cymodoce Gray” (Burmeister, 1867, p. 306). According to Burmeister (1867), these two skulls were considered similar to the holotype skull of T. cymodoce illustrated in plate 19 of Gray (1846). The location of collection of one of these two skulls was apparently unknown, but the other was collected in the Uruguay River, north of the city Paysandú, Uruguay (Burmeister, 1867). Some years later, when Gray added the type locality Uruguay River in Gray (1871, p. 74), he described it as “Inhab. River Uragua. Mus. Buenos Ayres.” Did Gray (1871) simply parrot Burmeister when he cataloged the collection at the Natural History Museum in London? Recently, Wang et al. (2021) conducted a morphological comparison between the holotype of T. cymodoce and bottlenose dolphin specimens from the wSA (T. t. truncatus and T. t. gephyreus). Their goal was to determine whether T. cymodoce represented the recently recognized coastal subspecies of the wSA, T. t. gephyreus Lahille, 1908, found between southern Brazil and northern Argentina. If that were the case, this newly recognized coastal subspecies, T. t. gephyreus, would need to pass through a nomenclatural change since the type name cymodoce (Gray, 1846) predates the name gephyreus Lahille, 1908 and hence has precedence. Results indicated that the morphological characters of T. cymodoce were more in line with the nominotypical subspecies (T. t. truncatus); therefore T. cymodoce does not represent the coastal subspecies in the wSA (Wang et al., 2021). Although these findings do not eliminate the Uruguay River as the type locality of T. cymodoce, they increase the support that Gray's locality was inaccurate. The nearshore and coastal waters of the wSA, including the Uruguay River, are known to be inhabited mainly by the coastal subspecies, T. t. gephyreus. The nominotypical subspecies (T. t. truncatus) has a more offshore distribution, and its occurrence in the specific region of the Uruguay River is not expected (Costa et al., 2016; Vermeulen et al., 2019). One way to attempt to clarify the provenance of T. cymodoce is through use of genetic data. The advance in molecular techniques has enabled the sampling of different types of biological material (e.g., bones, teeth) of specimens ranging from a few to hundreds of years old (Austin & Melville, 2006; Strutzenberger et al., 2012; van Helden et al., 2002), and it can potentially retrieve genetic characters that are unique to specimens of a specific region or species (Baker et al., 2003; Strutzenberger et al., 2012; Tautz et al., 2003). Mitochondrial DNA (mtDNA) has been effectively used in historical DNA analyses (e.g., Austin & Melville, 2006; Puillandre et al., 2012; Stuart & Fritz, 2008) and has become a useful tool for species identification and delimitations in different taxa (e.g., Dalebout et al., 2003; Miller et al., 2016; Strutzenberger et al., 2012; Stuart & Fritz, 2008; Taylor et al., 2017). More specifically, the mtDNA control region has been commonly used for species identification of closely related groups of cetaceans (e.g., Baker et al., 2003), and, in Tursiops, its haplotype sequences can be useful in distinguishing species, ecotypes (coastal vs. offshore) and populations in different oceanographic regions (e.g., Costa et al., 2021, 2022; Louis et al., 2014; Lowther-Thieleking et al., 2015; Rosel et al., 2009; Wang et al., 1999). By comparing the mtDNA control region sequence of the holotype T. cymodoce with a set of sequences retrieved worldwide, we can potentially identify the provenance of this nominal species. Bone powder from the holotype T. cymodoce (NHMUK GERM.355a) was obtained using a drill (Terratek FUT18V01–3, 18 V) and drill bits of 2.5–3.5 mm to create a small hole in the lower portion of the right occipital condyle (extracting 30.9 mg of powder). Sterilized drill bits, lab spatulas, and collection plates and tubes were used during sampling of the bone powder. To minimize risk of contamination during sampling, we initially drilled the surface of the bone and discarded this powder. A new sterile drill bit was then used in the open small hole to collect the powder from inside the bone for DNA extraction. The powder was demineralized with rotation in 950 μl of EDTA (0.5 M, pH 8.0) at room temperature for approximately 18 hr without changing the solution. This demineralization step was followed by DNA extraction using the QIAamp DNA Investigator (QIAGEN) kit following the manufacturer's instructions for hard tissue extraction with modifications as in Rosel et al. (2021). The extraction was performed in an exclusive trace DNA laboratory. A negative control was used in the extraction and carried through all PCR and sequencing steps. The 5′ end of the mtDNA control region was amplified using two overlapping fragments with primer pairs L15824 (Rosel et al., 1999) and H16081_Turs (the CR3new primer in Costa et al., 2021, which was modified from the primer H16081 in Vollmer et al., 2011 to improve amplification in Tursiops) and L16061 (Tolley & Rosel, 2006) and H16265 (Rosel et al., 1999). PCR was performed in 50 μl reactions containing 20 mM Tris–HCl (pH 8.4), 50 mM KCl, 1.5 mM MgCl2, 150 μM dNTPs, 2.5 U Taq DNA polymerase (Invitrogen), 0.12 mg/ml bovine serum albumin (BSA; Sigma-Aldrich), 0.3 μM of each primer, and 5 μl of DNA. The PCR profile was: 95°C for 30 s, followed by 45 cycles of 30 s at 95°C, 50°C, and 72°C, with final extension of 72°C for 7 min. The PCR products were purified using SureClean Plus (Bioline Reagents) and sequenced in both directions using an Applied Biosystems (ABI) BigDye Terminator v.1.1 cycle sequencing kit and an ABI 3500 Genetic Analyzer. Forward and reverse reads of each fragment were edited using Geneious Prime 2021.2 (https://www.geneious.com), and consensus sequences of the two reads were created then assembled to produce one continuous sequence of the 5′ end of the mtDNA control region. We obtained sequence for a 365 base-pair (bp) fragment of the mtDNA control region from the Tursiops cymodoce holotype. We initially compared this sequence with 37 haplotypes of a 353 bp fragment of the mtDNA control region found in the two bottlenose dolphin subspecies from the wSA (T. t. gephyreus: n = 11; T. t. truncatus: n = 25; and one shared haplotype between the subspecies; Costa et al., 2021). This led us to observe seven nucleotide differences between T. cymodoce and both wSA subspecies (Table 1), indicating it is unlikely to represent either of these subspecies. We then used BLASTN (http://blast.ncbi.nlm.nih.gov/Blast.cgi) with the 365 bp consensus sequence to extend our sequence comparison across a broader geographic range of sequences available in the public database GenBank. This comparison led us to observe a 100% match over 3301 bp between the holotype T. cymodoce sequence and the haplotype BurruCR7 (GenBank accession number JN571469 from Charlton-Robb et al., 2011). This haplotype sequence is considered unique to “T. australis” specimens (Burrunan dolphins) endemic to the coastal waters of southeast Australia, more specifically Victoria and Tasmania (Charlton-Robb et al., 2006, 2011). We also performed a maximum likelihood phylogenetic analysis using 317 bp of the 365 bp fragment of mtDNA control region and Tursiops spp. sequences from around the world, including haplotype BurruCR7 (the 317 bp fragment of the haplotype sequence (GenBank accession number: JN571469) is 100% identical to the sequence obtained for T. cymodoce). The maximum likelihood tree was built using IQ-Tree web server (Trifinopoulos et al., 2016) with ultrafast bootstrap (UFBoot; Minh et al., 2013) and Shimodaira-Hasegawa-like approximate likelihood ratio test (SH-aLRT; Guindon et al., 2010) as described in Costa et al. (2022). The best evolutionary model for DNA substitution selected using jModel Test and BIC was the HKY model (Hasegawa et al., 1985) with invariant sites and gamma. Tree visualization was performed using FigTree v.1.4.3 (http://tree.bio.ed.ac.uk/software/figtree/) with a bootstrap (UFBoot) cut-off value of 80%. In this tree (Figure 1), the haplotype BurruCR7, and hence our identical T. cymodoce sequence, clusters with other sequences attributed to Burrunan dolphins from either Victorian or Tasmanian waters in a clade with a high bootstrap value (UFBoot = 100), within a clade of common bottlenose dolphins (see also Figure S1 and Table S1). Noteworthy, no specimens or DNA from Australia or from the Burranan type of bottlenose dolphins had ever been handled before in the facility where the DNA analysis of T. cymodoce was conducted. Although there is some overlap in ranges of the four measurements used among the three groups of Tursiops spp. (Table 2), our results indicated that the cranial measurements of the T. cymodoce type specimen were usually above the mean obtained for T. aduncus and below the mean for “T. australis” and T. truncatus (Table 2). Considering that the skull of the holotype is of a young specimen2 (see also Costa et al., 2016; Flower 1883; True 1889), this result likely rules out T. aduncus as the source of this skull but does not allow us to distinguish between T. truncatus and the Burrunan dolphin form. Further support for ruling out T. aduncus is that the premaxillary “pinch” at approximately one-third rostral length (dorsal view) cited as a useful morphological characteristic that distinguish T. aduncus from other bottlenose dolphins (see fig. 8 in Wang et al., 2000) was not observed in T. cymodoce. For the three cranial features following Charlton-Robb et al. (2011), the pterygoids of T. cymodoce were partially broken, but the remaining structure suggests it bore small pterygoids (Figure 2B). We also observed a smooth transition in the maxilla-premaxilla area (Figure 2A), and although there was residual dried soft tissue attached to the proximal end of the palatines, it was possible to observe a more elongated triangular-shaped palatine (Figure 2B). These cranial features are in line with what was described for the Burrunan dolphin skulls in comparison to T. truncatus from southeast Australia, indicating morphological similarities between T. cymodoce and Burrunan dolphins. However, it is important to highlight that Jedensjö et al. (2020) did not find these differences to be fully diagnostic when comparing a bigger sample size from Australian waters. Through a detailed study of the cranial morphology (based on two-dimensional measurements and three-dimensional geometric morphometrics) of Tursiops spp. from Australian waters, the authors demonstrated that the Burrunan dolphin clusters among T. truncatus samples (Jedensjö et al., 2020). These morphological findings reveal a different result than genetics studies (based on nuclear and mtDNA), which distinguished the Burrunan dolphin from other common and Indo-Pacific bottlenose dolphins (Moura et al., 2013, 2020). In our study, we observed 100% identity over a 330 bp fragment of the mtDNA control region between T. cymodoce and a published Burrunan dolphin haplotype. Although we used a short fragment, which can sometimes be problematic due to low resolutions in intraspecific comparisons, Burrunan dolphin haplotypes are very distinct within Tursiops, even when considering the entire mitogenome (Moura et al., 2013). Thus, even if the morphological data do not provide definitive diagnostic characters to differentiate Burrunan dolphins from other T. truncatus, the genetic finding alone is strong enough to indicate that the holotype T. cymodoce presents significant similarities with Burrunan dolphins from southeast Australia. These results also show congruence with the original description of this holotype as being obtained during the voyage of H.M.S. Erebus and H.M.S. Terror: southeast Australia was in the route of the expedition. The expedition arrived in southern Tasmania (Hobart harbor) in August 1840, remaining in these waters until November 1840 when it departed to explore Antarctic waters. The expedition then returned again to Tasmania in April 1841, staying there until July 1841, when it traveled north to Sydney before heading back south to the Antarctic (Palin, 2018). In conclusion, we question the type locality provided by Gray (1871) for the holotype specimen T. cymodoce (Gray, 1846). The International Code on Zoological Nomenclature provides Recommendation 76A.2, wherein it states: “a statement of a type locality that is found to be erroneous should be corrected” (ICZN, 1999). Wang et al. (2021) previously ruled out the possibility that T. cymodoce represented the coastal subspecies of bottlenose dolphins in the western South Atlantic, T. t. gephyreus. Further, our morphological and genetic comparisons suggest the holotype T. cymodoce is similar to Burrunan dolphins and was probably collected in southeast Australia (e.g., Tasmania or southeast Australia mainland) rather than Uruguay. Therefore, here, we make our case to amend the type locality of T. cymodoce to southeast Australia following the Recommendation 76A.2 of the ICZN. Given this change, future nomenclatural evaluations of Tursiops spp. in southeast Australia need to consider the nominal species T. cymodoce (Gray, 1846), as this name predates the nominal T. australis Charlton-Robb et al., 2011. Ana Costa: Conceptualization; formal analysis; investigation; methodology; project administration; supervision; validation; visualization; writing – original draft; writing – review and editing. Lynsey Wilcox: Conceptualization; data curation; formal analysis; investigation; methodology; validation; writing – original draft; writing – review and editing. Richard Sabin: Data curation; resources; writing – review and editing. Patricia Rosel: Conceptualization; data curation; investigation; project administration; resources; supervision; writing – review and editing. We are grateful to Jasmin Perera (Loans and Exhibitions Coordinator, Natural History Museum, London) and Kelly Robertson (SWFSC – La Jolla) for their immense help with CITES and shipment of the bone powder sample of the type specimen Tursiops cymodoce from the United Kingdom to the United States. Our acknowledgments are also extended to Keith Mullin (SEFSC – Pascagoula) and three reviewers for suggestions on this manuscript. The scientific results and conclusions, in addition to any views or opinions expressed herein, are those of the authors and do not necessarily reflect those of NOAA or the Department of Commerce. The haplotype of Tursiops cymodoce found in this study was deposited in GenBank under the accession number OQ595207. Appendix S1. Supplementary Information Please note: The publisher is not responsible for the content or functionality of any supporting information supplied by the authors. Any queries (other than missing content) should be directed to the corresponding author for the article.
更多查看译文
AI 理解论文
溯源树
样例
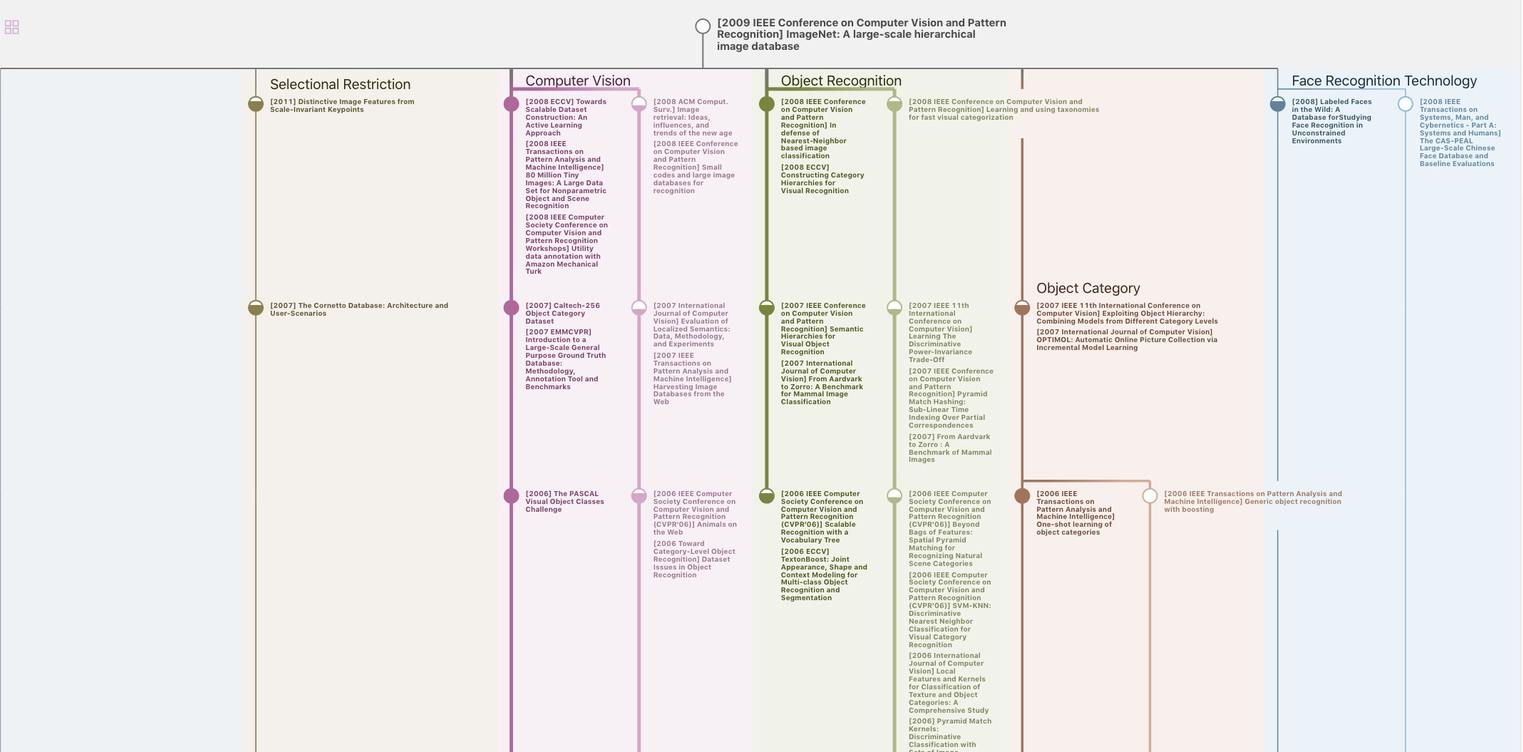
生成溯源树,研究论文发展脉络
Chat Paper
正在生成论文摘要