Understanding the Degradation Mechanisms of Lithium Ion Batteries Using in-Situ Multi-Scale Diffraction Techniques
ECS Meeting Abstracts(2022)
摘要
Advanced Li-ion batteries adopting new cathode chemistries are required for the successful widespread transition to electric vehicles (EVs) and renewable energy sources, aiming for high energy density, long cycle life, and good rate capability. Commercial candidates for EV batteries include Ni-rich Li(NixMnyCo1−x-y)O2 (NMC) cathodes, with Ni:Mn:Co ratios of 8:1:1 (NMC811) and higher. These are favored because of their high specific capacity (~ 200 mAh g-1)and reduced cobalt content. Despite all of the advantages, these materials suffer from a range of degradation modes, many of which are associated with the redox and crystallographic behavior at high states of charge. In particular, Ni-rich cathodes suffer from several limitations, such as rapid capacity fade in comparison to NMC stoichiometries with lower Ni content. In addition, they also have a lower onset voltage for oxygen release and subsequent surface reconstruction leading to the formation of spinel and rock salt phases which impede (de)lithiation and therefore the achievable capacity of the cell.1 Crystallographic properties of electrode materials are intrinsically linked to the electrochemical performance of the cell. NMC materials suffer from anisotropic changes in the crystal structure during cycling which induces strain and leads to issues such as crack formation, expediting degradation. One method to tackle capacity fade is to switch to single-crystal morphologies (particle size 1-3 μm) which have better mechanical stability than conventional polycrystalline morphologies (secondary agglomerate particles ~ 10 μm made up of primary particles which are 100 nm – 1 μm in size) and have less propensity to form extensive rock-salt layers. It is thought that the single-crystal morphology helps to reduce stress in the material as the anisotropic stress in polycrystalline cathodes is concentrated at grain boundaries. However, there is still a limited understanding of the subtle mechanistic differences between the two materials during cycling.2 A multi-scale approach is required to gain a more comprehensive understanding of the degradation mechanisms at play and how they initiate and propagate. In this work, synchrotron diffraction methods were employed at the crystal, particle and cell scale using a variety of techniques including in-situ Bragg Coherent Diffraction Imaging (BCDI), 3D-XRD and operando high-resolution XRD. Intra-particle, inter-particle and electrode level heterogeneities were observed during cycling, both in pristine and aged samples. It is believed that these heterogeneities accelerate the loss of performance at the cell level by inducing crack formation which can then be observed in X-ray computed tomography data acquired in simultaneous lab studies. The overarching goal of these investigations is to add to the understanding of complex degradation mechanisms for Ni-rich layered transition metal oxide cathodes, ultimately aiding in the informed development of future battery electrode materials. References: 1] Xu, C. et al., Phase Behaviour during Electrochemical Cycling of Ni‐Rich Cathode Materials for Li‐Ion Batteries. Adv. Energy Mater. 2021, 11, 2003404. 2] Yin, S. et al., Fundamental and solutions of microcracks in Ni-rich layered oxide cathode materials of lithium-ion batteries. Nano Energy, 2021, 83, 105854.
更多查看译文
关键词
lithium degradation batteries,degradation batteries,degradation mechanisms,in-situ,multi-scale
AI 理解论文
溯源树
样例
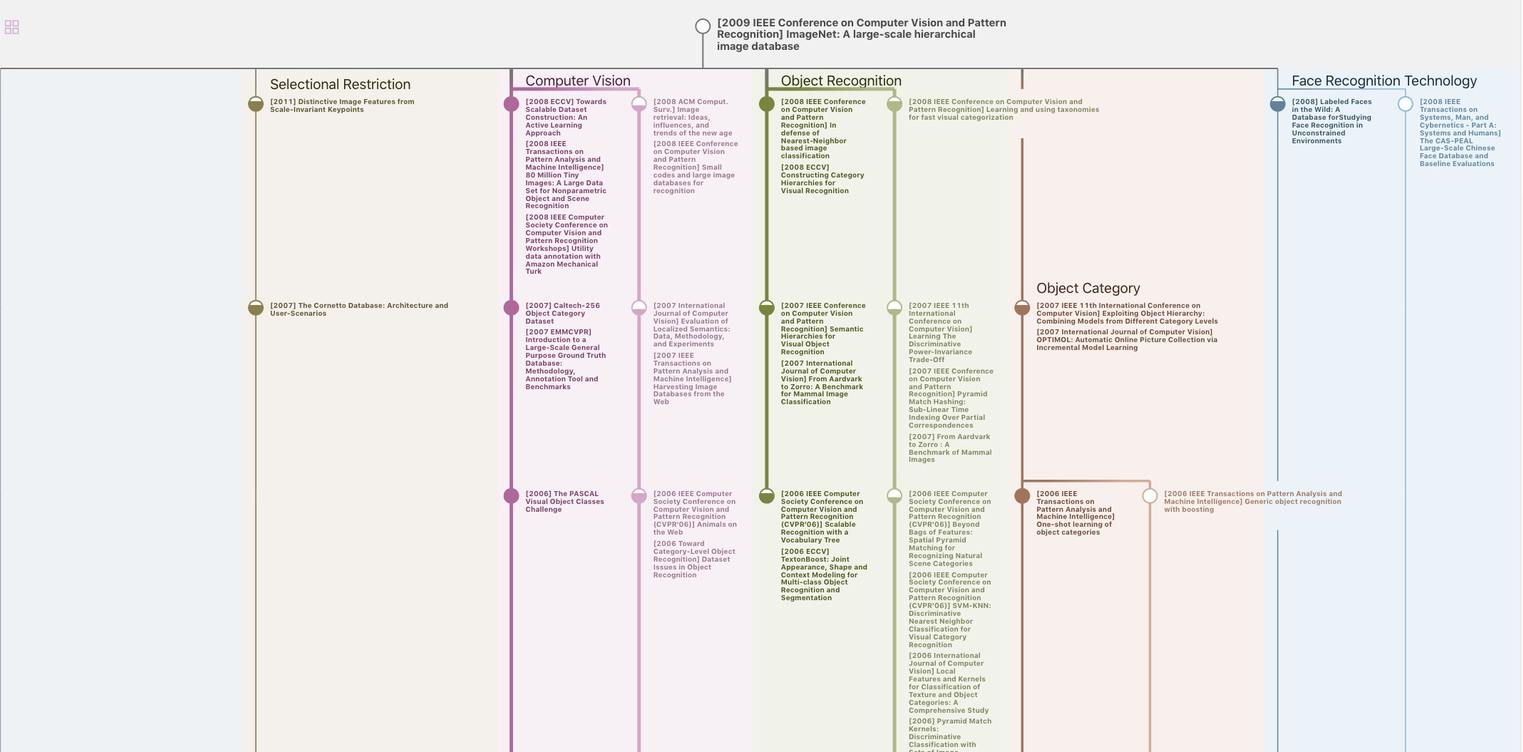
生成溯源树,研究论文发展脉络
Chat Paper
正在生成论文摘要