Tissue architecture in tumor initiation and progression
Trends in Cancer(2022)
摘要
Interplay between cellular, molecular, and mechanical factors shapes tumor morphology.Tumor architecture has genetic, epigenetic, and phenotypic effects on cancer cells.Primary tumor architecture informs cancer progression.Cytoskeletal architecture remodels before metastatic invasion.Carcinoma cells invade as single cells upon epithelial-to-mesenchymal transition or as epithelial clusters.The architecture and topography of the tumor impacts on therapy delivery and efficacy. The 3D architecture of tissues bearing tumors impacts on the mechanical microenvironment of cancer, the accessibility of stromal cells, and the routes of invasion. A myriad of intrinsic and extrinsic forces exerted by the cancer cells, the host tissue, and the molecular and cellular microenvironment modulate the morphology of the tumor and its malignant potential through mechanical, biochemical, genetic, and epigenetic cues. Recent studies have investigated how tissue architecture influences cancer biology from tumor initiation and progression to distant metastatic seeding and response to therapy. With a focus on carcinoma, the most common type of cancer, this review discusses the latest discoveries on how tumor architecture is built and how tissue morphology affects the biology and progression of cancer cells. The 3D architecture of tissues bearing tumors impacts on the mechanical microenvironment of cancer, the accessibility of stromal cells, and the routes of invasion. A myriad of intrinsic and extrinsic forces exerted by the cancer cells, the host tissue, and the molecular and cellular microenvironment modulate the morphology of the tumor and its malignant potential through mechanical, biochemical, genetic, and epigenetic cues. Recent studies have investigated how tissue architecture influences cancer biology from tumor initiation and progression to distant metastatic seeding and response to therapy. With a focus on carcinoma, the most common type of cancer, this review discusses the latest discoveries on how tumor architecture is built and how tissue morphology affects the biology and progression of cancer cells. Tissue architecture is dependent on tensional homeostasis that is necessary for organ function [1.Latorre E. et al.Active superelasticity in three-dimensional epithelia of controlled shape.Nature. 2018; 563: 203-208Crossref PubMed Scopus (118) Google Scholar]. When tumors arise and grow, they alter the organized morphology of tissues, creating aberrant tensional forces and inducing changes in the mechanical microenvironment. The 3D morphology of the resulting lesion and the associated aberrant mechanics influence the biology of cancer cells and surrounding tissues. Recently, several groups have focused on studying the etiology of different tumor architectures, their evolution throughout progression, and their prognostic value [2.Messal H.A. et al.Tissue curvature and apicobasal mechanical tension imbalance instruct cancer morphogenesis.Nature. 2019; 566: 126-130Crossref PubMed Scopus (60) Google Scholar, 3.Fiore V.F. et al.Mechanics of a multilayer epithelium instruct tumour architecture and function.Nature. 2020; 585: 433-439Crossref PubMed Scopus (38) Google Scholar, 4.van Royen M.E. et al.Three-dimensional microscopic analysis of clinical prostate specimens.Histopathology. 2016; 69: 985-992Crossref PubMed Scopus (51) Google Scholar]. This review discusses the recent literature evaluating the relevance of tissue architecture in tumor biology. The 3D architecture of tumor host tissues and malignant lesions is driven by mechanical traits and is a source of mechanical and functional intratumor heterogeneity. Diverse architectural properties of tissues, including their geometry, confinement, and fluidity, exert different mechanical stimuli on cancer cells within a tumor [5.Mohammadi H. Sahai E. Mechanisms and impact of altered tumour mechanics.Nat. Cell Biol. 2018; 20: 766-774Crossref PubMed Scopus (106) Google Scholar]. The disruption of tissue architecture at the onset of tumorigenesis is conditioned by the molecular drivers of transformation. Bona fide cancer drivers such as RAS oncogenes (HRAS, NRAS, and KRAS) cause loss of tensional homeostasis and reorganize tissues by altering local actomyosin contractility. In non-malignant mammary MCF-10A cells, stress fibers are localized in the apical region and contribute to the stiffness of the cell. In malignant mammary cells (MCF-7 and MDA-MB-231), stress fibers localize basally [6.Calzado-Martin A. et al.Effect of actin organization on the stiffness of living breast cancer cells revealed by peak-force modulation atomic force microscopy.ACS Nano. 2016; 10: 3365-3374Crossref PubMed Scopus (139) Google Scholar]. Upon transformation with oncogenic HRAS, non-malignant MCF-10A mammary cells lose their epithelial monolayer organization and aggregate into 3D structures in vitro. This occurs by reduction of cellular adhesion and decreased traction forces to the substrate, together with disrupted cytoskeletal polarization [7.Nyga A. et al.Oncogenic RAS instructs morphological transformation of human epithelia via differential tissue mechanics.Sci. Adv. 2021; 7eabg6467Crossref PubMed Scopus (1) Google Scholar]. Epithelial cells of the lung airways, hepatic ducts, and exocrine pancreas present a physiological apical polarization of actin and phosphorylated myosin. Upon transformation, constitutively active KRAS triggers the inhibition of phosphatases that maintain this actomyosin polarity, and leads to the basal accumulation of actomyosin cortex-related components including integrins and mechanoregulators [2.Messal H.A. et al.Tissue curvature and apicobasal mechanical tension imbalance instruct cancer morphogenesis.Nature. 2019; 566: 126-130Crossref PubMed Scopus (60) Google Scholar]. Thus, the local loss of cytoskeletal polarity in transformed cells contributes to the malignant cellular morphologies that in turn result in the characteristic architecture of early lesions (Figure 1). Throughout tumor progression, cell–cell interactions and tissue fluidity determine the individual cellular geometry [8.Atia L. et al.Geometric constraints during epithelial jamming.Nat. Phys. 2018; 14: 613-620Crossref PubMed Scopus (97) Google Scholar]. In MCF10A spheroids, the cytoplasmic and nuclear volume of cells varies between the core and periphery [9.Han Y.L. et al.Cell swelling, softening and invasion in a three-dimensional breast cancer model.Nat. Phys. 2020; 16: 101-108Crossref PubMed Scopus (86) Google Scholar], and different force magnitude levels are at play in these two regions, suggesting that local confinements throughout a tumor contribute to intratumor cellular heterogeneity. In the tumor margins, confinement by the extracellular matrix (ECM) plays a key role in cancer progression. Cancer cell confinement together with reduced cell–cell and cell–ECM adhesions regulate tumor unjamming transitions (increase of tissue fluidity) and promote invasion [10.Ilina O. et al.Cell–cell adhesion and 3D matrix confinement determine jamming transitions in breast cancer invasion.Nat. Cell Biol. 2020; 22: 1103-1115Crossref PubMed Scopus (86) Google Scholar]. Conversely, an increase in collagen concentration provokes tissue jamming and prevents invasion [11.Kang W. et al.A novel jamming phase diagram links tumor invasion to non-equilibrium phase separation.iScience. 2021; 24103252Abstract Full Text Full Text PDF Scopus (7) Google Scholar]. Under artificial confinement in vitro, HRASV12 transformed cells present a proliferation advantage. Although they are softer in intermitotic periods, their rigidity increases during mitosis, surpassing that of healthy cells, and thereby allows correct chromosome segregation without mitotic arrest [12.Matthews H.K. et al.Oncogenic signaling alters cell shape and mechanics to facilitate cell division under confinement.Dev. Cell. 2020; 52: 563-573Abstract Full Text Full Text PDF PubMed Scopus (29) Google Scholar]. Hence the mechanical heterogeneity driven by the tumor composition and architecture plays an important role in dictating cancer cell shape and competitive proliferation. The different local forces created by the tumor architecture have further consequences on the biology and behavior of cancer cells. In morphologically identical spherical colonies of breast cancer cells, traction stresses distribute stochastically, and a single cell can alter the organization and mechanics at the multicellular level [13.Schaumann E.N. et al.Force localization modes in dynamic epithelial colonies.Mol. Biol. Cell. 2018; 29: 2835-2847Crossref PubMed Scopus (14) Google Scholar]. Conversely, mouse and human cancer cell lines cultured in a variety of matrix shapes show tension at the matrix–media interface of curved geometrical traits. Increased local tension induces the expression of cancer stem cell markers and modulates cell shape, adhesion, and signaling [14.Lee J. et al.Interfacial geometry dictates cancer cell tumorigenicity.Nat. Mater. 2016; 15: 856-862Crossref PubMed Scopus (123) Google Scholar]. Tumor growth and shape, conditioned by mechanical tension and spatial restrictions among other factors, affect the diversification of genetic subclones in clear cell renal carcinoma, demonstrating crosstalk between cellular and architectural intratumor heterogeneity that impacts on cancer evolution [15.Fu X. et al.Spatial patterns of tumour growth impact clonal diversification in a computational model and the TRACERx renal study.Nat. Ecol. Evol. 2022; 6: 88-102Crossref PubMed Scopus (4) Google Scholar]. The use of spatial transcriptomics and single-cell RNA sequencing in human pancreatic ductal adenocarcinoma (PDAC) allowed the correlation of transcriptomic signatures and stromal enrichment with spatially isolated architectures within a tumor [16.Moncada R. et al.Integrating microarray-based spatial transcriptomics and single-cell RNA-seq reveals tissue architecture in pancreatic ductal adenocarcinomas.Nat. Biotechnol. 2020; 38: 333-342Crossref PubMed Scopus (202) Google Scholar]. The aberrant tissue architecture upon transformation alone could contribute to heterogeneity and malignancy through chromosome instability. Native tissue architecture and integrin function are necessary for proper chromosome segregation [17.Knouse K.A. et al.Chromosome segregation fidelity in epithelia requires tissue architecture.Cell. 2018; 175: 200-211Abstract Full Text Full Text PDF PubMed Scopus (69) Google Scholar], and cancer-induced disruption of epithelial architecture may lead to chromosome instability. Tumors of similar etiology or cell of origin often present different morphologies from early tumorigenesis that later affect their progression. The drivers of these different morphologies are starting to be elucidated, and, as in normal tissue developmental processes, these may depend on the organization and geometry of the naïve epithelium before transformation [18.Gjorevski N. et al.Tissue geometry drives deterministic organoid patterning.Science. 2022; 375eaaw9021Crossref PubMed Scopus (24) Google Scholar]. In the pancreas, the heterogeneous geometry of the pancreatic duct determines early morphogenesis of PDAC precursor lesions. Transformation in ducts with a diameter of 17 μm or above leads to the formation of endophytic lesions that grow into the duct lumen. The higher curvature of narrower ducts forces early tumors to grow exophytically into the surrounding parenchyma. Through their higher exposure to the non-cancerous tissue environment, exophytic lesions interact with protumorigenic cancer-associated fibroblasts (CAFs) more efficiently and display increased tumor cell dissemination [2.Messal H.A. et al.Tissue curvature and apicobasal mechanical tension imbalance instruct cancer morphogenesis.Nature. 2019; 566: 126-130Crossref PubMed Scopus (60) Google Scholar]. Strikingly, the actomyosin cortical perturbations are similar among cells in both lesion types, but lead to different tissue morphological outcomes owing to the disparate geometries of large- and small-diameter ducts and the cells within (Figure 1). In the skin, non-invasive basal cell carcinoma (BCC) forms bud-like lesions, whereas invasive squamous cell carcinoma (SCC) forms folds. This key architectural difference is explained by the assembly and stiffness of the basement membrane (BM). BCCs accelerate the assembly of the BM, creating a softer BM that is more resilient to tension. SCCs decrease BM assembly and exert higher tensional forces on it, facilitating rupture and invasion beyond [3.Fiore V.F. et al.Mechanics of a multilayer epithelium instruct tumour architecture and function.Nature. 2020; 585: 433-439Crossref PubMed Scopus (38) Google Scholar]. Thus, the spatial position of the tumor-originating cell in the normal tissue epithelium influences key aspects of lesion biology through an interplay between cell behavior and external geometric cues (Figure 1). Although driver mutations modulate cancer cell behavior and shape, local forces exerted by cancer cells and the geometry of the tissue before transformation condition tumor morphology, and the tumor-adjacent molecular and cellular microenvironment further crucially shapes tumor morphology. The tumor microenvironment is the ensemble of ECM molecules and non-transformed cells that surround cancer cells. Cancer cells secrete matrix modifiers [19.Jia H. et al.The tumor cell-secreted matricellular protein WISP1 drives pro-metastatic collagen linearization.EMBO J. 2019; 38e101302Crossref Scopus (15) Google Scholar] and exert forces [20.Mark C. et al.Collective forces of tumor spheroids in three-dimensional biopolymer networks.Elife. 2020; 9e51912Google Scholar,21.Szulczewski J.M. et al.Directional cues in the tumor microenvironment due to cell contraction against aligned collagen fibers.Acta Biomater. 2021; 129: 96-109Crossref PubMed Scopus (7) Google Scholar] to expand in restricted spaces and break through mechanical barriers. The architecture of ECM fibers and the cellular microenvironment can physically facilitate or restrict invasion. The biophysical characteristics of the tumor stroma have important implications in tumor morphology and cancer cell behavior (Figure 1). A stiffer stroma can foster invasion, which in part may come from the promotion of cancer cell interaction with the surrounding endothelium [22.Reid S.E. et al.Tumor matrix stiffness promotes metastatic cancer cell interaction with the endothelium.EMBO J. 2017; 36: 2373-2389Crossref PubMed Scopus (98) Google Scholar]. On the other hand, high ECM deposition stiffens the stroma and reduces pore sizes, leading to confinement and reduced tissue fluidity which may limit cancer cell proliferation. For example, PDAC lesions grow slower in their stiffer acellular primary site than as metastatic lesions in the liver, with reduced stroma [23.Jiang H. et al.Pancreatic ductal adenocarcinoma progression is restrained by stromal matrix.J. Clin. Invest. 2020; 130: 4704-4709Crossref PubMed Scopus (45) Google Scholar]. The ECM is an acellular array of macromolecules that function as a scaffold for maintaining the architecture of the tissue. Tumors have aberrant ECMs because tumor cells, CAFs, and other cells of the tumor microenvironment secrete components that alter the physicochemical characteristics of the matrix. The ECM is viscoelastic and it can also undergo irreversible deformations [24.Mohammadi H. et al.Inelastic behaviour of collagen networks in cell–matrix interactions and mechanosensation.J. R. Soc. Interface. 2015; 1220141074Crossref PubMed Scopus (51) Google Scholar]. Forces exerted by tumor growth alone can cause breaking of weak crosslinks and untangling of fibers [25.Chaudhuri O. et al.Effects of extracellular matrix viscoelasticity on cellular behaviour.Nature. 2020; 584: 535-546Crossref PubMed Scopus (376) Google Scholar]. Cancer cells can modulate the architecture of the ECM by exerting pulsating forces and contracting against collagen fibers, inducing anisotropy [20.Mark C. et al.Collective forces of tumor spheroids in three-dimensional biopolymer networks.Elife. 2020; 9e51912Google Scholar,21.Szulczewski J.M. et al.Directional cues in the tumor microenvironment due to cell contraction against aligned collagen fibers.Acta Biomater. 2021; 129: 96-109Crossref PubMed Scopus (7) Google Scholar]. Similarly, CAFs create forces that align fibers, creating anisotropic paths that facilitate cancer cell migration [26.Park D. et al.Extracellular matrix anisotropy is determined by TFAP2C-dependent regulation of cell collisions.Nat. Mater. 2020; 19: 227-238Crossref PubMed Scopus (55) Google Scholar]. The BM is a thin sheet of ECM that provides essential structural support to epithelial, mesothelial, and endothelial tissues, and constitutes a physical barrier for immune infiltration and tumor invasion. The BM is permeable to nutrients and is hyperelastic. Upon experimental pressure application, the BM was discovered to have a non-linear stiffening behavior, making it resistant to instability, breaching, or softening under mechanical stress [27.Li H. et al.Nonlinear elasticity of biological basement membrane revealed by rapid inflation and deflation.Proc. Natl. Acad. Sci. U. S. A. 2021; 118e2022422118Google Scholar]. BM stiffness is a key determinant of distant metastasis. Netrin-4 induces openings of laminin node complexes and softens the BM. Although this creates pores in the BM, the softening increases its resilience to growing tumors and reduces metastasis. The netrin-4/laminin ratio determines the stiffness of the mammary gland BM. A higher ratio results in lower stiffness and decreased invasion [28.Reuten R. et al.Basement membrane stiffness determines metastases formation.Nat. Mater. 2021; 20: 892-903Crossref PubMed Scopus (32) Google Scholar]. Recent evidence shows that the relationship between BM and tumors is more complex than was previously anticipated because tumors can produce their own BM [29.Morgner J. et al.A Lamb1Dendra2 mouse model reveals basement membrane-producing origins and dynamics in PyMT breast tumors.Dev. Cell. 2021; (Published online September 30, 2021)https://papers.ssrn.com/sol3/papers.cfm?abstract_id=3933999Google Scholar]. In vitro, CAFs can drive the invasion of single cells or collective migration by directly interacting with cancer cells through N-cadherin/E-cadherin heterotypic adhesions. CAFs exert pulling forces on the cancer cells away from 3D spheroids, inducing invasion [30.Labernadie A. et al.A mechanically active heterotypic E-cadherin/N-cadherin adhesion enables fibroblasts to drive cancer cell invasion.Nat. Cell Biol. 2017; 19: 224-237Crossref PubMed Scopus (380) Google Scholar]. In addition, CAFs can alter the integrity of the BM by exerting physical forces, making it permissive for tumor cell invasion, as shown in coculture experiments with colon cancer cell lines [31.Glentis A. et al.Cancer-associated fibroblasts induce metalloprotease-independent cancer cell invasion of the basement membrane.Nat. Commun. 2017; 8: 924Crossref PubMed Scopus (164) Google Scholar]. Conversely, adipose stromal fibroblasts stiffen the ECM by deposition of fiber proteins such as collagen and fibronectin [32.Seo B.R. et al.Obesity-dependent changes in interstitial ECM mechanics promote breast tumorigenesis.Sci. Transl. Med. 2015; 7301ra130Crossref Scopus (176) Google Scholar]. CAFs induce epithelial-to-mesenchymal transition (EMT) and proliferation of PDAC cells through TGF-β secretion. This leads to a heterogeneous composition of tumor cell types (proliferative, EMT, neither or both), allowing the classification in eight architecture 'units' that can coexist within the same tumor [33.Ligorio M. et al.Stromal microenvironment shapes the intratumoral architecture of pancreatic cancer.Cell. 2019; 178: 160-175Abstract Full Text Full Text PDF PubMed Scopus (227) Google Scholar]. CAFs also aid breast cancer growth in an architecture-dependent manner via secretion of IL-6, which induces local hepcidin expression and iron retention that are characteristic of breast cancer. Interestingly, this mechanism has only been observed in vivo and in organoids, and not in 2D culture, suggesting that the 3D architecture of the transformed epithelium is required [34.Blanchette-Farra N. et al.Contribution of three-dimensional architecture and tumor-associated fibroblasts to hepcidin regulation in breast cancer.Oncogene. 2018; 37: 4013-4032Crossref PubMed Scopus (27) Google Scholar]. Epithelial malignancies involve mechanical crosstalk between the expanding lesion and the surrounding healthy cells. Local transformation by KRASV12 in Xenopus laevis embryos leads to actomyosin hypercontractility, creating a radial anisotropic tension around the lesions and inducing division of contiguous wild-type cells [35.Moruzzi M. et al.Generation of anisotropic strain dysregulates wild-type cell division at the interface between host and oncogenic tissue.Curr. Biol. 2021; 31: 3409-3418Abstract Full Text Full Text PDF PubMed Scopus (2) Google Scholar]. In mouse skin, tissue straining through self-inflating gels boosts cell proliferation in the absence of tumors [36.Aragona M. et al.Mechanisms of stretch-mediated skin expansion at single-cell resolution.Nature. 2020; 584: 268-273Crossref PubMed Scopus (51) Google Scholar]. Hence the tension exerted by an expanding tumor affects the tissue shape and can induce proliferation of surrounding healthy cells. Extrusion of transformed cells is an epithelial defense mechanism against cancer that involves increased lateral interfacial actomyosin contractility [37.Bielmeier C. et al.Interface contractility between differently fated cells drives cell elimination and cyst formation.Curr. Biol. 2016; 26: 563-574Abstract Full Text Full Text PDF PubMed Scopus (83) Google Scholar] (Figure 2). However, cancer-induced stiffening of the surrounding ECM drives reorganization of the cytoskeleton of healthy cells including perinuclear localization of filamin that impedes extrusion [38.Pothapragada S.P. et al.Matrix mechanics regulates epithelial defence against cancer by tuning dynamic localization of filamin.Nat. Commun. 2022; 13: 218Crossref PubMed Scopus (2) Google Scholar]. The basal myoepithelium of glandular organs such as the mammary gland and prostate impairs the preinvasive-to-invasive transition of transformed luminal cells. Genetic perturbations that affect the function of the myoepithelium increase the risk of invasive carcinomas [39.Ding L. et al.Perturbed myoepithelial cell differentiation in BRCA mutation carriers and in ductal carcinoma in situ.Nat. Commun. 2019; 10: 4182Crossref PubMed Scopus (21) Google Scholar]. Live imaging of mammary tumor 3D cultures has revealed that the myoepithelium not only functions as a physical barrier against invasive phenotypes of cancer cells but also dynamically impairs invasion by interacting with cells undergoing EMT, exerting pulling forces and returning them to the luminal region upon escape [40.Sirka O.K. et al.Myoepithelial cells are a dynamic barrier to epithelial dissemination.J. Cell Biol. 2018; 217: 3368-3381Crossref PubMed Scopus (11) Google Scholar]. The mechanisms inducing the forces that myoepithelial cells exert on cancer cells of the luminal epithelial layer remain to be investigated. Most recently, morphometric quantifications comparing the myoepithelium of patients with ductal carcinoma in situ have shown that progressors have a thicker continuous myoepithelium [41.Risom T. et al.Transition to invasive breast cancer is associated with progressive changes in the structure and composition of tumor stroma.Cell. 2022; 185: 299-310Abstract Full Text Full Text PDF PubMed Scopus (14) Google Scholar], perhaps in response to aggressive luminal phenotypes. The recent success of immunotherapy against cancer has focused the attention of researchers on the immune microenvironment. Different immune cells play distinct pro- and antitumorigenic functions. Inflammation induced by immune cells can change the biophysical properties of a tumor. Furthermore, by modulating the architecture of the ECM, immune cells impact on the architecture and invasive routes of tumors. Natural killer (NK) cells interact with B16 melanoma cells through Ncr1 receptor–Ncr1 ligand interaction. This induces IFN-γ secretion by NK cells which promotes tumor cells to express the ECM protein fibronectin 1. Increased fibronectin 1 builds a benign architecture of the primary tumor that impedes metastasis [42.Glasner A. et al.NKp46 receptor-mediated interferon-gamma production by natural killer cells increases fibronectin 1 to alter tumor architecture and control metastasis.Immunity. 2018; 48: 107-119Abstract Full Text Full Text PDF PubMed Scopus (86) Google Scholar]. At the cellular level the aberrant cytoskeletal architecture of cancer cells can be detected by cytotoxic cells. Myocardin-related transcription factors can enhance the rigidity of the cytoskeleton of cancer cells and their invasiveness in the absence of immune cells. However, cytotoxic T lymphocytes and NK cells efficiently detect and eliminate cancer cells with increased rigidity [43.Tello-Lafoz M. et al.Cytotoxic lymphocytes target characteristic biophysical vulnerabilities in cancer.Immunity. 2021; 54: 1037-1054Abstract Full Text Full Text PDF PubMed Scopus (24) Google Scholar]. Consistently, inducing stiffening of the cancer cell plasma membrane by depleting cholesterol enhances T cell cytotoxicity [44.Ley K. et al.Cancer-cell stiffening via cholesterol depletion enhances adoptive T-cell immunotherapy.Nat. Biomed. Eng. 2021; 5: 1411-1425Crossref PubMed Scopus (10) Google Scholar]. Macrophages create crosslinking in the ECM by secreting lysyl oxidase (LOX) enzymes that stiffen the stroma and induce cell invasion and poor prognosis in patients with aggressive breast cancer [45.Maller O. et al.Tumour-associated macrophages drive stromal cell-dependent collagen crosslinking and stiffening to promote breast cancer aggression.Nat. Mater. 2021; 20: 548-559Crossref PubMed Scopus (45) Google Scholar]. In zebrafish, macrophages and neutrophils were shown to span breaches in the BM to access tumors of the epidermis. The cells have protumorigenic activity, and might aid invasion by creating breaches in the BM [46.van den Berg M.C.W. et al.Proteolytic and opportunistic breaching of the basement membrane zone by immune cells during tumor initiation.Cell Rep. 2019; 27: 2837-2846Abstract Full Text Full Text PDF PubMed Scopus (19) Google Scholar]. Chemotherapy has been shown to affect cancer cell behavior by modulating the activity of immune cells. After paclitaxel chemotherapy, CD8+ T cells have shown to remodel the ECM of lungs via LOX expression and the deposition of collagen and laminin, aiding the metastatic seeding of breast cancer cells [47.Haj-Shomaly J. et al.T cells promote metastasis by regulating extracellular matrix remodeling following chemotherapy.Cancer Res. 2022; 82: 278-291Crossref PubMed Scopus (7) Google Scholar]. These recent discoveries manifest the cell- and context-dependent role of the immune microenvironment in enhancing or impairing tumorigenesis and invasion by modifying mechanical traits. Cancer cells acquire invasive traits throughout primary tumor evolution and metastasize through the lymphatic and blood vasculature to distant organs. In this section we differentiate between two types of carcinoma invasion based on tissue and cell architecture. Upon EMT, cancer cells undergo total remodeling of their cytoskeletal architecture and can abandon the tissue of origin as single cells [48.Yang J. et al.Guidelines and definitions for research on epithelial–mesenchymal transition.Nat. Rev. Mol. Cell Biol. 2020; 21: 341-352Crossref PubMed Scopus (504) Google Scholar]. Epithelial cells or cells that undergo partial EMT can invade tissues and vasculature as clusters, partially preserving their epithelial organization (Figure 2). EMT is induced by oncogenes that remodel the cytoskeleton (as discussed in the preceding text) and by the physical properties of the tumor such as interstitial fluid pressure [49.Piotrowski-Daspit A.S. et al.Interstitial fluid pressure regulates collective invasion in engineered human breast tumors via snail, vimentin, and E-cadherin.Integr. Biol. (Camb.). 2016; 8: 319-331Crossref PubMed Google Scholar] and forces exerted by the stroma. A higher ECM stiffness correlates with the invasive phenotype of cells of different cancer types. Non-malignant MCF10A breast cells behave like invasive cancer cells in stiff matrices [50.Chaudhuri O. et al.Extracellular matrix stiffness and composition jointly regulate the induction of malignant phenotypes in mammary epithelium.Nat. Mater. 2014; 13: 970-978Crossref PubMed Scopus (513) Google Scholar]. In endometrial cancer cells, EGF induces EpCAM proteolysis, nuclear translocation of its intracellular region, and interaction with LEF1. These molecules act as transcription coactivators and upregulate the key EMT regulators TWIST1, ZEB1, and SNAI1. Atomic force microscopy (AFM) experiments revealed that cells treated with EGF are both softer and less adhesive as a result of EMT and loss of membrane EpCAM, respectively [51.Hsu Y.T. et al.EpCAM-regulated transcription exerts influences on nanomechanical properties of endometrial cancer cells that promote epithelial-to-mesenchymal transition.Cancer Res. 2016; 76: 6171-6182Crossref PubMed Scopus (34) Google Scholar]. BAR proteins sense and generate membrane curvature and are inhibited physiologically in epithelial cells [52.Mim C. Unger V.M. Membrane curvature and its generation by BAR proteins.Trends Biochem. Sci. 2012; 37: 526-533Abstract Full Text Full Text PDF PubMed Scopus (183) Google Scholar]. The plasma membrane tension is maintained by membrane-to-cortex attachment proteins ezrin, radixin, and moesin (ERM) in healthy
更多查看译文
关键词
tissue architecture,mechanics,tumorigenesis,tumor progression
AI 理解论文
溯源树
样例
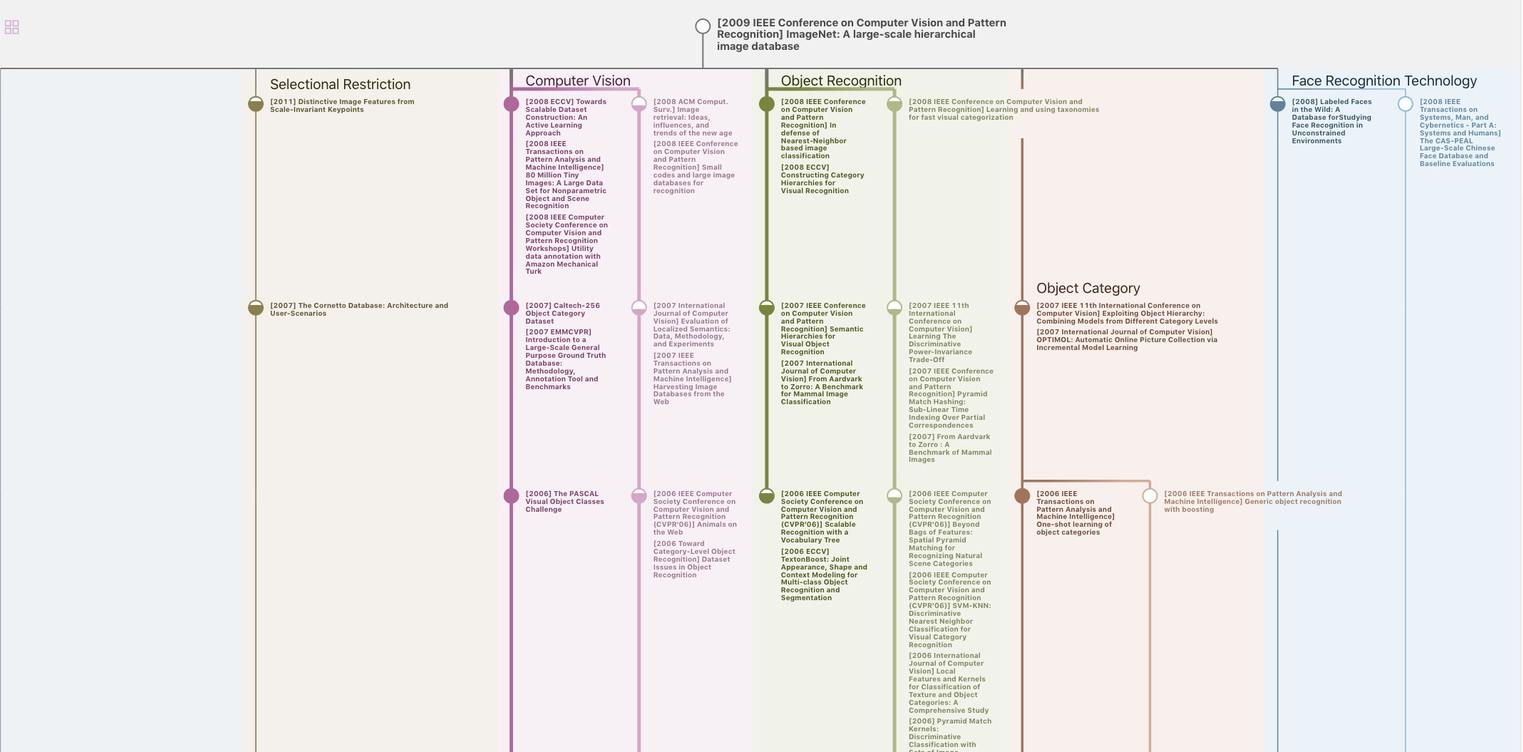
生成溯源树,研究论文发展脉络
Chat Paper
正在生成论文摘要