Structural and Genetic Analysis of the PknB Sensor Domain
semanticscholar(2016)
摘要
Monitoring the environment with serine/threonine protein kinases is critical for growth and survival of Mycobacterium tuberculosis, a devastating human pathogen. Protein Kinase B (PknB) is a trans-membrane serine/threonine protein kinase that acts as an essential regulator of mycobacterial growth and division. The PknB extracellular domain (ECD) consists of four repeats homologous to penicillin-binding protein and serine/threonine kinase associated (PASTA) domains, and binds fragments of peptidoglycan. These properties suggest that PknB activity is modulated by ECD binding to peptidoglycan substructures, however the molecular mechanisms underpinning PknB regulation remain unclear. In this study, we report structural and genetic characterization of the PknB ECD. We determined the crystal structures of overlapping ECD fragments at near atomic resolution, built a model of the full ECD, and discovered a region on the Cterminal PASTA domain that has the properties of a ligand-binding site. Hydrophobic interaction between this surface and a bound molecule of citrate was observed in a crystal structure. Our genetic analyses in Mycobacterium tuberculosis showed that nonfunctional alleles were produced either by deletion of any of single PASTA domain or by mutation of individual conserved residues lining the putative ligand-binding surface of the Cterminal PASTA repeat. These results define two distinct structural features necessary for PknB signal transduction, a fully extended ECD and a conserved, membrane-distal putative ligandbinding site. INTRODUCTION In addition to the classical bacterial histidine kinases, Mycobacterium tuberculosis (Mtb) encodes eleven eukaryotic-like serine/threonine protein kinases (STPK) with functions in growth, virulence, and persistence (1). While over 250 STPK phosphorylation targets have been identified, the environmental cues that regulate STPKs have remained largely unknown (2). Nonetheless, PknB has emerged as a strong candidate for coordination of cell wall peptidoglycan remodeling with multiple intracellular pathways. Following activation induced by formation of an asymmetric homodimer (3), PknB phosphorylates proteins involved in peptidoglycan synthesis, the tricarboxylic acid cycle, cell division, and the extracellular stress response (4,5), consistent with its proposed role in cell-wall homeostasis. PknB contains an intracellular N-terminal kinase domain followed by a 50-residue linker, a single transmembrane helix and a peptidoglycaninteracting ECD that comprises four PASTA Structural and Genetic Analysis of the PknB Sensor Domain 2 repeats, termed PASTA1-4. This architecture is found throughout Firmicutes and Actinobacteria with homologs reported in Streptococcus (6), Staphilococcus (7), Bacillus (8), and Corynebacterium (9), although the number of PASTA domains is variable and additional immunoglobulin-like domains are present in ECDs of some of the homologs (10). Phylogenetic analysis revealed position-dependent evolution indicative of specialization of individual PASTA domains and found that the PASTA domain composition of a particular protein is specific to each genus (11). In Bacillus subtilis, Protein Kinase C, a PknB homolog, is dispensable for normal growth but is required for peptidoglycan fragment-induced spore reactivation, with the PASTA domains determining peptidoglycan species specificity (8). In mycobacteria, PknB deletion is lethal, knockdown results in elongated cells, and overexpression produces round bulbous cells (12). Direct interaction of muropeptides with PknB ECD is similarly dependent on fine chemical modifications and requires at least one sugar and three peptide residues of the peptidoglycan monomer (13). The ECD determines polar localization of PknB, a step that may be required for proper activation of kinase signaling. Structure determination of PknB ECD using nuclear magnetic resonance, revealed an extended conformation of the PASTA domains in solution and suggested a ligand-dependent dimerization activation mechanism (14). Allelic substitution experiments with a C-terminal truncation series reported by Chawla et al. indicated that all alleles lacking PASTA4 were nonfunctional (15). Conversely, overexpression of a C-terminal fragment of PknB that included its transmembrane domain and the ECD suppressed mycobacterial growth in a PASTA4-dependent manner (16). These genetic data argued for the presence of ligand binding site in PASTA4. In this study we determined five X-ray crystal structures of the PknB ECD allowing us to build a complete atomic model from the two largest overlapping fragments. Binding of a citrate molecule to a highly conserved hydrophobic groove on PASTA4 in one of the structures identified a potential ligandbinding site. Allelic substitution experiments in M. tuberculosis confirmed the functional significance of this PASTA4 surface and revealed an unexpected requirement for each of the PASTA domains for proper PknB function. RESULTS In order to determine the atomic structure of the PknB ECD and to create a platform for investigation of ligand binding, we expressed and purified multiple constructs corresponding to individual PASTA domains and domain combinations. Proteins representing PASTA1-2, PASTA2-4 and PASTA3-4 (domains are numbered from Nto C-terminus) as well as the full ECD were purified by glutathione affinity chromatography and gel filtration, and were tested for crystallization against a panel of sparse-matrix screens. While diffraction quality crystals of the full ECD were never obtained, several shorter constructs yielded crystals that diffracted to ~2 Å resolution. The PASTA4 structure was determined at 2.0 Å resolution using single-wavelength anomalous dispersion of Zn-bound protein. Zn ions were present in the crystallization solution and 9 were found in the refined structure (Table 1, Figure 1A). Of these, two were essential to forming crystal contacts. As reported previously, the defining structural features of a PASTA domain include a ψ-loop, which has one face exposed to the solvent, and an amphipathic α-helix that is nested on the concave side of this β-structure. In the case of PknB PASTA4, the β1-β2 loop is 13 residues long and includes a 310 helix (residues 597-599). An L512M mutant of PASTA3 labeled with selenomethionine (Figure 1B) was purified and crystallized as an alternate approach for solving the phase problem. However, the phases were determined using molecular replacement with PASTA4 and the structure refined, also at 2.0 Å resolution, against data obtained at 1.116 Å wavelength, away from Se edge. The overall fold of PASTA3 domain is very similar to PASTA4 except that the loop between β-1 and β-2 is only 8 residues long and does not form a 310 helix. We found that in order to achieve crystallization of multi-domain constructs it was necessary to trim the unstructured N-terminal extensions. Our Structural and Genetic Analysis of the PknB Sensor Domain 3 starting set of constructs contained N-terminal GHM linker residues that remained following TEV cleavage. When several of these failed to crystallize, we took advantage of the naturally occurring glycine residues of PASTA domains 2 (G423) and 3 (G491) to clone constructs that incorporated these residues as part of the TEV recognition site (ENLYFQ|G). While TEV cleavage efficiency was decreased, the resulting processed constructs contained no linker artifacts and crystallized readily. In the case of PASTA1-2, an analogous glycine (G355) would have given a construct containing an unstructured N-terminus. Instead, a single glycine linker residue was cloned upstream of amino acid V360 creating a construct that crystallized well. PASTA3-4 at 2.0 Å, PASTA2-4 at 2.2 Å, and lastly PASTA1-2 at 1.8 Å resolution (Figure 1C, 1D, 1E) were solved by molecular replacement with previously determined structures. Of these, PASTA2-4 proved the most challenging as search with the PASTA3-4 structure failed, likely due to a difference in inter-domain angles. Instead, sequential searches with the individual PASTA domains produced phases that resulted in an interpretable electron density map. The complete atomic model of the PknB ECD was then constructed based on this structure and the overlapping PASTA1-2 fragment (Figure 2A). The relationship between PASTA domains in the full ECD is that observed previously for this family of sensor kinases. The PASTA domains of PknB stack top to bottom rather than side to side resulting in an extended conformation. The linkers between PASTA domains are short and well ordered in every structure presented. To achieve bending angles much greater than those observed, the domains might have to undergo partial unfolding. Such conformations are thus likely to be transient. In order to map the functionally significant surfaces in the PknB ECD, we undertook bioinformatic analysis. The pairwise identity of individual PASTA domains to each other is low, around 30%. In contrast, full-length alignment of PknB orthologs from the Mycobacterium family reveals a striking degree of surface conservation in the ECD that focuses on PASTA4 and the linker region between PASTA1 and PASTA2 (Figure 2B). Of these two regions only the conserved patch in PASTA4 has partially hydrophobic character (Figure 2C). The conserved region in PASTA4 domain was bound to a molecule of citric acid in the PASTA 24 structure (Figure 3) suggesting it is a ligandbinding site. This citrate-binding site is formed between helix α1 and strand β1. Citrate contacts include side chain atoms of residues Phe624, Asn601 and Lys589. The bottom of the groove in which the citrate molecule is bound is hydrophobic and in addition to Phe624 contains partially exposed conserved tryptophan (Trp571), a typical feature of protein binding sites (Figure 3). The citrate molecule is positioned to interact with this site through its hydrophobic face with all three charged carboxylic groups and the hydroxy
更多查看译文
AI 理解论文
溯源树
样例
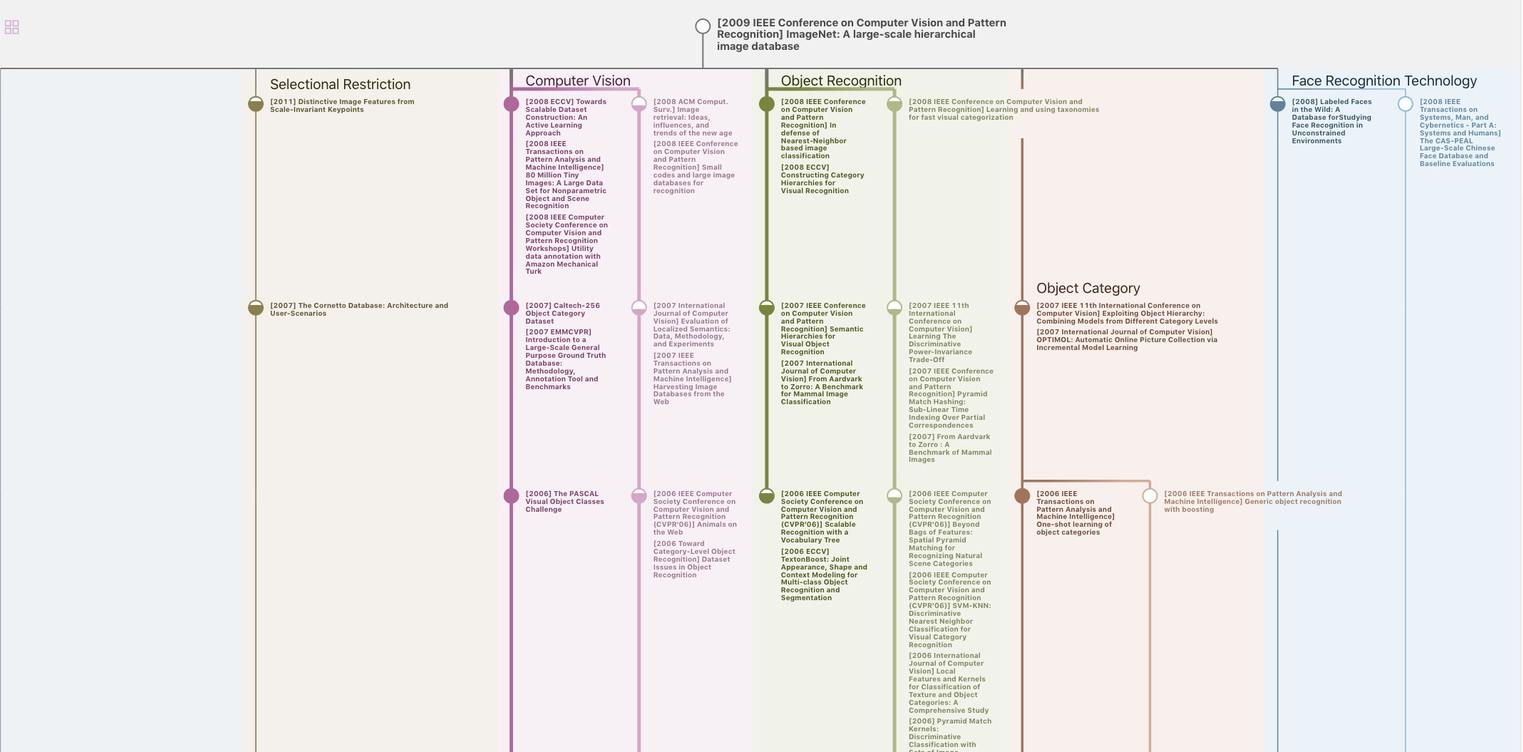
生成溯源树,研究论文发展脉络
Chat Paper
正在生成论文摘要